RAB23 coordinates early osteogenesis by repressing FGF10-pERK1/2 and GLI1
- PMID: 32662771
- PMCID: PMC7423339
- DOI: 10.7554/eLife.55829
RAB23 coordinates early osteogenesis by repressing FGF10-pERK1/2 and GLI1
Abstract
Mutations in the gene encoding Ras-associated binding protein 23 (RAB23) cause Carpenter Syndrome, which is characterized by multiple developmental abnormalities including polysyndactyly and defects in skull morphogenesis. To understand how RAB23 regulates skull development, we generated Rab23-deficient mice that survive to an age where skeletal development can be studied. Along with polysyndactyly, these mice exhibit premature fusion of multiple sutures resultant from aberrant osteoprogenitor proliferation and elevated osteogenesis in the suture. FGF10-driven FGFR1 signaling is elevated in Rab23-/-sutures with a consequent imbalance in MAPK, Hedgehog signaling and RUNX2 expression. Inhibition of elevated pERK1/2 signaling results in the normalization of osteoprogenitor proliferation with a concomitant reduction of osteogenic gene expression, and prevention of craniosynostosis. Our results suggest a novel role for RAB23 as an upstream negative regulator of both FGFR and canonical Hh-GLI1 signaling, and additionally in the non-canonical regulation of GLI1 through pERK1/2.
Keywords: Craniosynostosis; GLI1; MAPK signaling; RAB23; RUNX2; developmental biology; medicine; mouse.
Plain language summary
In many animals, the skull is made of several separate bones that are loosely joined during childhood and only fuse into one piece when the animal stops growing. A genetic disease called Carpenter syndrome causes the bones of the skull to fuse early in life, stopping it from growing correctly. Carpenter syndrome is often caused by changes to the gene responsible for making a protein called RAB23. RAB23 helps move other molecules and cell components between different parts of the cell, and is therefore involved in a number of cellular processes. Previous studies suggest that RAB23 has a role in many parts of the body during development. Yet, it is unclear which cells in the skull depend on RAB23 activity and how this protein is controlled. To answer this question, Hasan et al. grew pieces of developing skull bones that had been taken from mice lacking the RAB23 protein in the laboratory. Examining these samples revealed that RAB23 is active in cells called osteoblasts that add new bone to the edge of each piece of the skull as it grows. Hasan et al. also found that RAB23 regulates two cellular signaling pathways – called the hedgehog pathway and the fibroblast growth factor pathway – that interact with one another and co-ordinate skull development. These findings show how RAB23 controls the growth and fusion of skull bones in developing animals. This could improve our understanding of the role RAB23 plays in other processes during development. It also sheds light on the mechanisms of Carpenter syndrome which may inform new approaches for treating patients.
© 2020, Hasan et al.
Conflict of interest statement
MH, MT, HM, RR, TM, DR No competing interests declared
Figures
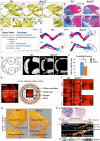
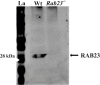
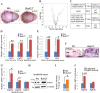
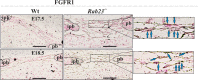
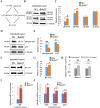
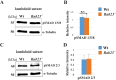
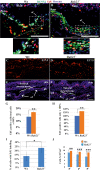
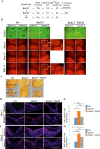
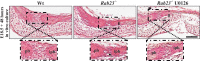
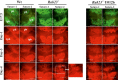
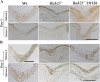
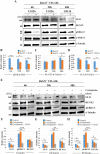
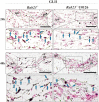
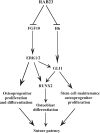
Similar articles
-
Rab23 negatively regulates Gli1 transcriptional factor in a Su(Fu)-dependent manner.Cell Signal. 2012 Jun;24(6):1222-8. doi: 10.1016/j.cellsig.2012.02.004. Epub 2012 Feb 18. Cell Signal. 2012. PMID: 22365972 Free PMC article.
-
RAB23 mutations in Carpenter syndrome imply an unexpected role for hedgehog signaling in cranial-suture development and obesity.Am J Hum Genet. 2007 Jun;80(6):1162-70. doi: 10.1086/518047. Epub 2007 Apr 18. Am J Hum Genet. 2007. PMID: 17503333 Free PMC article.
-
Rab23 regulates differentiation of ATDC5 chondroprogenitor cells.J Biol Chem. 2008 Apr 18;283(16):10649-57. doi: 10.1074/jbc.M706795200. Epub 2008 Jan 23. J Biol Chem. 2008. PMID: 18218620 Free PMC article.
-
Rab23 and developmental disorders.Rev Neurosci. 2018 Nov 27;29(8):849-860. doi: 10.1515/revneuro-2017-0110. Rev Neurosci. 2018. PMID: 29727300 Review.
-
Rab23's genetic structure, function and related diseases: a review.Biosci Rep. 2017 Mar 2;37(2):BSR20160410. doi: 10.1042/BSR20160410. Print 2017 Apr 30. Biosci Rep. 2017. PMID: 28104793 Free PMC article. Review.
Cited by
-
Population genetic structure analysis and identification of backfat thickness loci of Chinese synthetic Yunan pigs.Front Genet. 2022 Nov 9;13:1039838. doi: 10.3389/fgene.2022.1039838. eCollection 2022. Front Genet. 2022. PMID: 36437945 Free PMC article.
-
Ciliary Signalling and Mechanotransduction in the Pathophysiology of Craniosynostosis.Genes (Basel). 2021 Jul 14;12(7):1073. doi: 10.3390/genes12071073. Genes (Basel). 2021. PMID: 34356089 Free PMC article. Review.
-
Embryology, Malformations, and Rare Diseases of the Cochlea.Laryngorhinootologie. 2021 Apr;100(S 01):S1-S43. doi: 10.1055/a-1349-3824. Epub 2021 Apr 30. Laryngorhinootologie. 2021. PMID: 34352899 Free PMC article.
-
Cranium growth, patterning and homeostasis.Development. 2022 Nov 15;149(22):dev201017. doi: 10.1242/dev.201017. Epub 2022 Nov 21. Development. 2022. PMID: 36408946 Free PMC article.
-
The clinical manifestations, molecular mechanisms and treatment of craniosynostosis.Dis Model Mech. 2022 Apr 1;15(4):dmm049390. doi: 10.1242/dmm.049390. Epub 2022 Apr 22. Dis Model Mech. 2022. PMID: 35451466 Free PMC article. Review.
References
-
- Al Alam D, El Agha E, Sakurai R, Kheirollahi V, Moiseenko A, Danopoulos S, Shrestha A, Schmoldt C, Quantius J, Herold S, Chao CM, Tiozzo C, De Langhe S, Plikus MV, Thornton M, Grubbs B, Minoo P, Rehan VK, Bellusci S. Evidence for the involvement of fibroblast growth factor 10 in lipofibroblast formation during embryonic lung development. Development. 2015;142:4139–4150. doi: 10.1242/dev.109173. - DOI - PMC - PubMed
-
- Bochukova EG, Roscioli T, Hedges DJ, Taylor IB, Johnson D, David DJ, Deininger PL, Wilkie AO. Rare mutations of FGFR2 causing apert syndrome: identification of the first partial gene deletion, and an alu element insertion from a new subfamily. Human Mutation. 2009;30:204–211. doi: 10.1002/humu.20825. - DOI - PubMed
Publication types
MeSH terms
Substances
Associated data
- Actions
Grants and funding
LinkOut - more resources
Full Text Sources
Molecular Biology Databases
Miscellaneous