Cryo-EM of human Arp2/3 complexes provides structural insights into actin nucleation modulation by ARPC5 isoforms
- PMID: 32661131
- PMCID: PMC7406314
- DOI: 10.1242/bio.054304
Cryo-EM of human Arp2/3 complexes provides structural insights into actin nucleation modulation by ARPC5 isoforms
Abstract
The Arp2/3 complex regulates many cellular processes by stimulating formation of branched actin filament networks. Because three of its seven subunits exist as two different isoforms, mammals produce a family of Arp2/3 complexes with different properties that may be suited to different physiological contexts. To shed light on how isoform diversification affects Arp2/3 function, we determined a 4.2 Å resolution cryo-EM structure of the most active human Arp2/3 complex containing ARPC1B and ARPC5L, and compared it with the structure of the least active ARPC1A-ARPC5-containing complex. The architecture of each isoform-specific Arp2/3 complex is the same. Strikingly, however, the N-terminal half of ARPC5L is partially disordered compared to ARPC5, suggesting that this region of ARPC5/ARPC5L is an important determinant of complex activity. Confirming this idea, the nucleation activity of Arp2/3 complexes containing hybrid ARPC5/ARPC5L subunits is higher when the ARPC5L N-terminus is present, thereby providing insight into activity differences between the different Arp2/3 complexes.
Keywords: Actin; Arp2/3; Cryo-EM; Cytoskeleton; Isoforms; Nucleation.
© 2020. Published by The Company of Biologists Ltd.
Conflict of interest statement
Competing interestsThe authors declare no competing or financial interests.
Figures
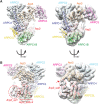
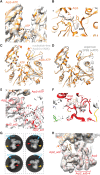
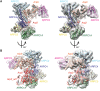
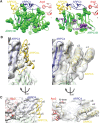
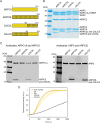
Similar articles
-
Nucleation, stabilization, and disassembly of branched actin networks.Trends Cell Biol. 2022 May;32(5):421-432. doi: 10.1016/j.tcb.2021.10.006. Epub 2021 Nov 23. Trends Cell Biol. 2022. PMID: 34836783 Free PMC article. Review.
-
Isoform diversity in the Arp2/3 complex determines actin filament dynamics.Nat Cell Biol. 2016 Jan;18(1):76-86. doi: 10.1038/ncb3286. Epub 2015 Dec 14. Nat Cell Biol. 2016. PMID: 26655834
-
ArpC5 isoforms regulate Arp2/3 complex-dependent protrusion through differential Ena/VASP positioning.Sci Adv. 2023 Jan 20;9(3):eadd6495. doi: 10.1126/sciadv.add6495. Epub 2023 Jan 20. Sci Adv. 2023. PMID: 36662867 Free PMC article.
-
Cryo-EM reveals the transition of Arp2/3 complex from inactive to nucleation-competent state.Nat Struct Mol Biol. 2020 Nov;27(11):1009-1016. doi: 10.1038/s41594-020-0481-x. Epub 2020 Aug 24. Nat Struct Mol Biol. 2020. PMID: 32839613 Free PMC article.
-
The stabilization of Arp2/3 complex generated actin filaments.Biochem Soc Trans. 2024 Feb 28;52(1):343-352. doi: 10.1042/BST20230638. Biochem Soc Trans. 2024. PMID: 38288872 Free PMC article. Review.
Cited by
-
CryoTRANS: predicting high-resolution maps of rare conformations from self-supervised trajectories in cryo-EM.Commun Biol. 2024 Aug 27;7(1):1058. doi: 10.1038/s42003-024-06739-9. Commun Biol. 2024. PMID: 39191900 Free PMC article.
-
Nucleation, stabilization, and disassembly of branched actin networks.Trends Cell Biol. 2022 May;32(5):421-432. doi: 10.1016/j.tcb.2021.10.006. Epub 2021 Nov 23. Trends Cell Biol. 2022. PMID: 34836783 Free PMC article. Review.
-
Cellular stiffness sensing through talin 1 in tissue mechanical homeostasis.Sci Adv. 2024 Aug 23;10(34):eadi6286. doi: 10.1126/sciadv.adi6286. Epub 2024 Aug 21. Sci Adv. 2024. PMID: 39167642 Free PMC article.
-
MICAL2 enhances branched actin network disassembly by oxidizing Arp3B-containing Arp2/3 complexes.J Cell Biol. 2021 Aug 2;220(8):e202102043. doi: 10.1083/jcb.202102043. Epub 2021 Jun 9. J Cell Biol. 2021. PMID: 34106209 Free PMC article.
-
ARPC5 isoforms and their regulation by calcium-calmodulin-N-WASP drive distinct Arp2/3-dependent actin remodeling events in CD4 T cells.Elife. 2023 May 10;12:e82450. doi: 10.7554/eLife.82450. Elife. 2023. PMID: 37162507 Free PMC article.
References
-
- Adams P. D., Afonine P. V., Bunkóczi G., Chen V. B., Davis I. W., Echols N., Headd J. J., Hung L.-W., Kapral G. J., Grosse-Kunstleve R. W. et al. (2010). Phenix: a comprehensive python-based system for macromolecular structure solution. Acta Crystallogr. D Biol. Crystallogr. 66, 213-221. 10.1107/S0907444909052925 - DOI - PMC - PubMed
-
- Brigida I., Zoccolillo M., Cicalese M. P., Pfajfer L., Barzaghi F., Scala S., Oleaga-Quintas C., Álvarez-Álvarez J. A., Sereni L., Giannelli S. et al. (2018). T-cell defects in patients with ARPC1B germline mutations account for combined immunodeficiency. Blood 132, 2362-2374. 10.1182/blood-2018-07-863431 - DOI - PMC - PubMed
Publication types
MeSH terms
Substances
Grants and funding
LinkOut - more resources
Full Text Sources