Doping bioactive elements into a collagen scaffold based on synchronous self-assembly/mineralization for bone tissue engineering
- PMID: 32637748
- PMCID: PMC7327760
- DOI: 10.1016/j.bioactmat.2020.06.005
Doping bioactive elements into a collagen scaffold based on synchronous self-assembly/mineralization for bone tissue engineering
Abstract
Pure collagen is biocompatible but lacks inherent osteoinductive, osteoimmunomodulatory and antibacterial activities. To obtain collagen with these characteristics, we developed a novel methodology of doping bioactive elements into collagen through the synchronous self-assembly/mineralization (SSM) of collagen. In the SSM model, amorphous mineral nanoparticles (AMN) (amorphous SrCO3, amorphous Ag3PO4, etc.) stabilized by the polyampholyte, carboxymethyl chitosan (CMC), and collagen molecules were the primary components under acidic conditions. As the pH gradually increased, intrafibrillar mineralization occurred via the self-adaptive interaction between the AMNs and the collagen microfibrils, which were self-assembling; the AMNs wrapped around the microfibrils became situated in the gap zones of collagen and finally transformed into crystals. Sr-doped collagen scaffolds (Sr-CS) promoted in vitro cell proliferation and osteogenic differentiation of rat bone marrow mesenchymal stromal cells (rBMSCs) and synergistically improved osteogenesis of rBMSCs by altering the macrophage response. Ag-doped collagen scaffolds (Ag-CS) exhibited in vitro antibacterial effects on S. aureus, as well as cell/tissue compatibility. Moreover, Sr-CS implanted into the calvarial defect of a rat resulted in improved bone regeneration. Therefore, the SSM model is a de novo synthetic strategy for doping bioactive elements into collagen, and can be used to fabricate multifunctional collagen scaffolds to meet the clinical challenges of encouraging osteogenesis, boosting the immune response and fighting severe infection in bone defects.
Keywords: Bioactive elements; Bone tissue engineering; Collagen scaffold; Synchronous self-assembly/mineralization.
© 2020 [The Author/The Authors].
Conflict of interest statement
The authors declare that they have no known competing financial interests or personal relationships that could have appeared to influence the work reported in this paper.
Figures
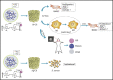
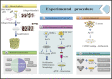
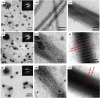
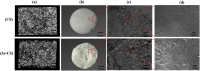
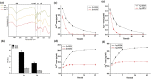
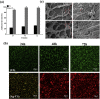
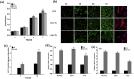
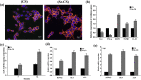
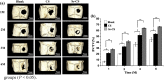
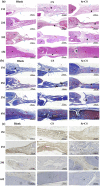
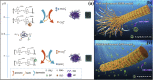
Similar articles
-
Synergistic intrafibrillar/extrafibrillar mineralization of collagen scaffolds based on a biomimetic strategy to promote the regeneration of bone defects.Int J Nanomedicine. 2016 May 12;11:2053-67. doi: 10.2147/IJN.S102844. eCollection 2016. Int J Nanomedicine. 2016. PMID: 27274235 Free PMC article.
-
3D-printed scaffolds with bioactive elements-induced photothermal effect for bone tumor therapy.Acta Biomater. 2018 Jun;73:531-546. doi: 10.1016/j.actbio.2018.04.014. Epub 2018 Apr 13. Acta Biomater. 2018. PMID: 29656075
-
Zero-order controlled release of BMP2-derived peptide P24 from the chitosan scaffold by chemical grafting modification technique for promotion of osteogenesis in vitro and enhancement of bone repair in vivo.Theranostics. 2017 Feb 27;7(5):1072-1087. doi: 10.7150/thno.18193. eCollection 2017. Theranostics. 2017. PMID: 28435449 Free PMC article.
-
Ag-loaded MgSrFe-layered double hydroxide/chitosan composite scaffold with enhanced osteogenic and antibacterial property for bone engineering tissue.J Biomed Mater Res B Appl Biomater. 2018 Feb;106(2):863-873. doi: 10.1002/jbm.b.33900. Epub 2017 Apr 17. J Biomed Mater Res B Appl Biomater. 2018. PMID: 28419693
-
Collagen functionalized bioactive nanofiber matrices for osteogenic differentiation of mesenchymal stem cells: bone tissue engineering.J Biomed Nanotechnol. 2014 Feb;10(2):287-98. doi: 10.1166/jbn.2014.1753. J Biomed Nanotechnol. 2014. PMID: 24738337
Cited by
-
Advances in Filament Structure of 3D Bioprinted Biodegradable Bone Repair Scaffolds.Int J Bioprint. 2021 Oct 13;7(4):426. doi: 10.18063/ijb.v7i4.426. eCollection 2021. Int J Bioprint. 2021. PMID: 34805599 Free PMC article. Review.
-
Biomaterial scaffolds in maxillofacial bone tissue engineering: A review of recent advances.Bioact Mater. 2023 Nov 10;33:129-156. doi: 10.1016/j.bioactmat.2023.10.031. eCollection 2024 Mar. Bioact Mater. 2023. PMID: 38024227 Free PMC article. Review.
-
Bioinspired mineralized collagen scaffolds for bone tissue engineering.Bioact Mater. 2020 Nov 16;6(5):1491-1511. doi: 10.1016/j.bioactmat.2020.11.004. eCollection 2021 May. Bioact Mater. 2020. PMID: 33294729 Free PMC article. Review.
-
Graphene-Oxide Porous Biopolymer Hybrids Enhance In Vitro Osteogenic Differentiation and Promote Ectopic Osteogenesis In Vivo.Int J Mol Sci. 2022 Jan 1;23(1):491. doi: 10.3390/ijms23010491. Int J Mol Sci. 2022. PMID: 35008918 Free PMC article.
-
Cyclical endometrial repair and regeneration: Molecular mechanisms, diseases, and therapeutic interventions.MedComm (2020). 2023 Dec 1;4(6):e425. doi: 10.1002/mco2.425. eCollection 2023 Dec. MedComm (2020). 2023. PMID: 38045828 Free PMC article. Review.
References
-
- Zhang W., Liao S.S., Cui F.Z. Hierarchical self-assembly of nano-fibrils in mineralized collagen. Chem. Mater. 2003;15(16):3221–3226.
-
- Du T., Niu X., Li Z., Li P., Feng Q., Fan Y. Crosslinking induces high mineralization of apatite minerals on collagen fibers. Int. J. Biol. Macromol. 2018;113:450–457. - PubMed
-
- Lin M., Liu H., Deng J., An R., Shen M., Li Y., Zhang X. Carboxymethyl chitosan as a polyampholyte mediating intrafibrillar mineralization of collagen via collagen/ACP self-assembly. J. Mater. Sci. Technol. 2019;35(9):1894–1905.
LinkOut - more resources
Full Text Sources
Research Materials
Miscellaneous