Silk fibroin as an additive for cell-free protein synthesis
- PMID: 32637668
- PMCID: PMC7320238
- DOI: 10.1016/j.synbio.2020.06.004
Silk fibroin as an additive for cell-free protein synthesis
Abstract
Cell-free systems contain many proteins and metabolites required for complex functions such as transcription and translation or multi-step metabolic conversions. Research into expanding the delivery of these systems by drying or by embedding into other materials is enabling new applications in sensing, point-of-need manufacturing, and responsive materials. Meanwhile, silk fibroin from the silk worm, Bombyx mori, has received attention as a protective additive for dried enzyme formulations and as a material to build biocompatible hydrogels for controlled localization or delivery of biomolecular cargoes. In this work, we explore the effects of silk fibroin as an additive in cell-free protein synthesis (CFPS) reactions. Impacts of silk fibroin on CFPS activity and stability after drying, as well as the potential for incorporation of CFPS into hydrogels of crosslinked silk fibroin are assessed. We find that simple addition of silk fibroin increased productivity of the CFPS reactions by up to 42%, which we attribute to macromolecular crowding effects. However, we did not find evidence that silk fibroin provides a protective effects after drying as previously described for purified enzymes. Further, the enzymatic crosslinking transformations of silk fibroin typically used to form hydrogels are inhibited in the presence of the CFPS reaction mixture. Crosslinking attempts did not impact CFPS activity, but did yield localized protein aggregates rather than a hydrogel. We discuss the mechanisms at play in these results and how the silk fibroin-CFPS system might be improved for the design of cell-free devices.
Keywords: CFPS; CFPS, Cell-free Protein Synthesis; Cell-free protein synthesis; Cell-free systems; HRP, horse radish peroxidase; Preservation; SEM, Scanning Electron Microscopy; SF, Silk Fibroin; Silk fibroin; sfGFP, superfolder green fluorescent protein.
Conflict of interest statement
The authors have no competing interests to declare.
Figures
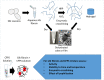
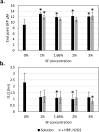
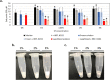
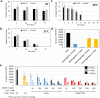
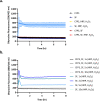
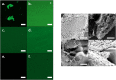
Similar articles
-
Impacts of Blended Bombyx mori Silk Fibroin and Recombinant Spider Silk Fibroin Hydrogels on Cell Growth.Polymers (Basel). 2021 Nov 29;13(23):4182. doi: 10.3390/polym13234182. Polymers (Basel). 2021. PMID: 34883685 Free PMC article.
-
Enzyme-Mediated Conjugation of Peptides to Silk Fibroin for Facile Hydrogel Functionalization.Ann Biomed Eng. 2020 Jul;48(7):1905-1915. doi: 10.1007/s10439-020-02503-2. Epub 2020 Apr 20. Ann Biomed Eng. 2020. PMID: 32314301 Free PMC article.
-
Silk protein-based hydrogels: Promising advanced materials for biomedical applications.Acta Biomater. 2016 Feb;31:17-32. doi: 10.1016/j.actbio.2015.11.034. Epub 2015 Nov 18. Acta Biomater. 2016. PMID: 26602821 Review.
-
Preparation and Characterization of Natural Silk Fibroin Hydrogel for Protein Drug Delivery.Molecules. 2022 May 25;27(11):3418. doi: 10.3390/molecules27113418. Molecules. 2022. PMID: 35684356 Free PMC article.
-
Processing, mechanical properties and bio-applications of silk fibroin-based high-strength hydrogels.Acta Biomater. 2021 Apr 15;125:57-71. doi: 10.1016/j.actbio.2021.02.018. Epub 2021 Feb 16. Acta Biomater. 2021. PMID: 33601067 Review.
Cited by
-
Multiscale design of cell-free biologically active architectural structures.Front Bioeng Biotechnol. 2023 Mar 28;11:1125156. doi: 10.3389/fbioe.2023.1125156. eCollection 2023. Front Bioeng Biotechnol. 2023. PMID: 37064226 Free PMC article.
-
Self-assembly of a multimeric genomic hydrogel via multi-primed chain reaction of dual single-stranded circular plasmids for cell-free protein production.iScience. 2023 Jun 10;26(7):107089. doi: 10.1016/j.isci.2023.107089. eCollection 2023 Jul 21. iScience. 2023. PMID: 37416467 Free PMC article.
-
Methodologies for preparation of prokaryotic extracts for cell-free expression systems.Synth Syst Biotechnol. 2020 Jul 30;5(4):252-267. doi: 10.1016/j.synbio.2020.07.006. eCollection 2020 Dec. Synth Syst Biotechnol. 2020. PMID: 32775710 Free PMC article. Review.
References
LinkOut - more resources
Full Text Sources