Investigating the roles of the conserved Cu2+-binding residues on Brucella FtrA in producing conformational stability and functionality
- PMID: 32623149
- PMCID: PMC7484176
- DOI: 10.1016/j.jinorgbio.2020.111162
Investigating the roles of the conserved Cu2+-binding residues on Brucella FtrA in producing conformational stability and functionality
Abstract
Brucella is a zoonotic pathogen requiring iron for its survival and acquires this metal through the expression of several high-affinity uptake systems. Of these, the newly discovered ferrous iron transporter, FtrABCD, is proposed to take part in ferrous iron uptake. Sequence homology shows that, FtrA, the proposed periplasmic ferrous-binding component, is a P19-type protein (a periplasmic protein from C. jejuni which shows Cu2+ dependent iron affinity). Previous structural and biochemical studies on other P19 systems have established a Cu2+ dependent Mn2+ affinity as well as formation of homodimers for these systems. The Cu2+ coordinating amino acids from these proteins are conserved in Brucella FtrA, hinting towards similar properties. However, there has been no experimental evidence, till date, establishing metal affinities and the possibility of dimer formation by Brucella FtrA. Using wild-type FtrA and Cu2+-binding mutants (H65A, E67A, H118A, and H151A) we investigated the metal affinities, folding stabilities, dimer forming abilities, and the molecular basis of the Cu2+ dependence for this P19-type protein employing homology modeling, analytical gel filtration, calorimetric, and spectroscopic methods. The data reported here confirm a Cu2+-dependent, low-μM Mn2+ (Fe2+ mimic) affinity for the wild-type FtrA. In addition, our data clearly show the loss of Mn2+ affinity, and the formation of less stable protein conformations as a result of mutating these conserved Cu2+-binding residues, indicating the important roles these residues play in producing a native and functional fold of Brucella FtrA.
Keywords: Brucella; Cu(2+)-binding mutant; Differential scanning calorimetry; FtrA; Homology model; Isothermal titration calorimetry.
Published by Elsevier Inc.
Figures
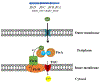
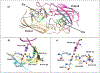
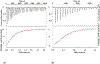
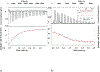
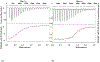
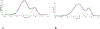
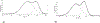
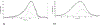
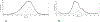
Similar articles
-
New insights into the mechanism of iron transport through the bacterial Ftr system present in pathogens.FEBS J. 2022 Oct;289(20):6286-6307. doi: 10.1111/febs.16476. Epub 2022 May 28. FEBS J. 2022. PMID: 35527501
-
The ferrous iron transporter FtrABCD is required for the virulence of Brucella abortus 2308 in mice.Mol Microbiol. 2013 Jun;88(6):1070-82. doi: 10.1111/mmi.12242. Epub 2013 May 5. Mol Microbiol. 2013. PMID: 23647104
-
Molecular structure and metal-binding properties of the periplasmic CopK protein expressed in Cupriavidus metallidurans CH34 during copper challenge.J Mol Biol. 2008 Jul 4;380(2):386-403. doi: 10.1016/j.jmb.2008.05.017. Epub 2008 May 15. J Mol Biol. 2008. PMID: 18533181
-
A review on bacterial redox dependent iron transporters and their evolutionary relationship.J Inorg Biochem. 2022 Apr;229:111721. doi: 10.1016/j.jinorgbio.2022.111721. Epub 2022 Jan 10. J Inorg Biochem. 2022. PMID: 35033753 Review.
-
The copper transporter (Ctr) family of Cu+ uptake systems.J Mol Microbiol Biotechnol. 2006;11(1-2):10-9. doi: 10.1159/000092815. J Mol Microbiol Biotechnol. 2006. PMID: 16825786 Review.
References
-
- Pappas G, Papadimitriou P, Akritidis N, Christou L and Tsianos EV, Lancet Infect. Dis, 2006, 6, 91. - PubMed
-
- Young EJ, in Principles and Practice of Infectious Diseases, ed. Mandell GL, Bennett JEand Dolin R, Elsevier Churchill Livingstone, Philadelphia PA, 6th ed, 2005, Part III, 2669.
Publication types
MeSH terms
Substances
Grants and funding
LinkOut - more resources
Full Text Sources