Regulation of Three Key Kinases of Brassinosteroid Signaling Pathway
- PMID: 32570783
- PMCID: PMC7352359
- DOI: 10.3390/ijms21124340
Regulation of Three Key Kinases of Brassinosteroid Signaling Pathway
Abstract
Brassinosteroids (BRs) are important plant growth hormones that regulate a wide range of plant growth and developmental processes. The BR signals are perceived by two cell surface-localized receptor kinases, Brassinosteroid-Insensitive1 (BRI1) and BRI1-Associated receptor Kinase (BAK1), and reach the nucleus through two master transcription factors, bri1-EMS suppressor1 (BES1) and Brassinazole-resistant1 (BZR1). The intracellular transmission of the BR signals from BRI1/BAK1 to BES1/BZR1 is inhibited by a constitutively active kinase Brassinosteroid-Insensitive2 (BIN2) that phosphorylates and negatively regulates BES1/BZR1. Since their initial discoveries, further studies have revealed a plethora of biochemical and cellular mechanisms that regulate their protein abundance, subcellular localizations, and signaling activities. In this review, we provide a critical analysis of the current literature concerning activation, inactivation, and other regulatory mechanisms of three key kinases of the BR signaling cascade, BRI1, BAK1, and BIN2, and discuss some unresolved controversies and outstanding questions that require further investigation.
Keywords: GSK3-like kinases; brassinosteroids; protein phosphatases; receptor-like kinases; somatic embryogenesis receptor-like kinases.
Conflict of interest statement
The authors declare no conflict of interest.
Figures
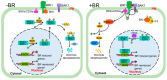
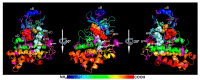
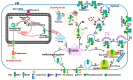
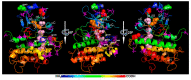
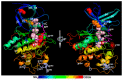
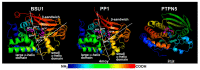
Similar articles
-
MYBL2 is a substrate of GSK3-like kinase BIN2 and acts as a corepressor of BES1 in brassinosteroid signaling pathway in Arabidopsis.Proc Natl Acad Sci U S A. 2012 Dec 4;109(49):20142-7. doi: 10.1073/pnas.1205232109. Epub 2012 Nov 19. Proc Natl Acad Sci U S A. 2012. PMID: 23169658 Free PMC article.
-
State-of-the-Art Technologies for Understanding Brassinosteroid Signaling Networks.Int J Mol Sci. 2020 Oct 31;21(21):8179. doi: 10.3390/ijms21218179. Int J Mol Sci. 2020. PMID: 33142942 Free PMC article. Review.
-
Two putative BIN2 substrates are nuclear components of brassinosteroid signaling.Plant Physiol. 2002 Nov;130(3):1221-9. doi: 10.1104/pp.102.010918. Plant Physiol. 2002. PMID: 12427989 Free PMC article.
-
Transcription factor HAT1 is phosphorylated by BIN2 kinase and mediates brassinosteroid repressed gene expression in Arabidopsis.Plant J. 2014 Jan;77(1):59-70. doi: 10.1111/tpj.12368. Epub 2013 Dec 3. Plant J. 2014. PMID: 24164091
-
The brassinosteroid signal transduction pathway.Cell Res. 2006 May;16(5):427-34. doi: 10.1038/sj.cr.7310054. Cell Res. 2006. PMID: 16699538 Free PMC article. Review.
Cited by
-
Glycogen synthase kinases in model and crop plants - From negative regulators of brassinosteroid signaling to multifaceted hubs of various signaling pathways and modulators of plant reproduction and yield.Front Plant Sci. 2022 Jul 15;13:939487. doi: 10.3389/fpls.2022.939487. eCollection 2022. Front Plant Sci. 2022. PMID: 35909730 Free PMC article. Review.
-
Dissection of canopy layer-specific genetic control of leaf angle in Sorghum bicolor by RNA sequencing.BMC Genomics. 2022 Feb 3;23(1):95. doi: 10.1186/s12864-021-08251-4. BMC Genomics. 2022. PMID: 35114939 Free PMC article.
-
Salicylic acid attenuates brassinosteroid signaling via protein de-S-acylation.EMBO J. 2023 Jul 3;42(13):e112998. doi: 10.15252/embj.2022112998. Epub 2023 May 22. EMBO J. 2023. PMID: 37211868 Free PMC article.
-
Assessment of Biological Activity of 28-Homobrassinolide via a Multi-Level Comparative Analysis.Int J Mol Sci. 2023 May 27;24(11):9377. doi: 10.3390/ijms24119377. Int J Mol Sci. 2023. PMID: 37298328 Free PMC article.
-
Rhizobia induce SYMRK endocytosis in Phaseolus vulgaris root hair cells.Planta. 2023 Mar 16;257(4):83. doi: 10.1007/s00425-023-04116-0. Planta. 2023. PMID: 36928335 Free PMC article.
References
-
- Nawaz F., Naeem M., Zulfiqar B., Akram A., Ashraf M.Y., Raheel M., Shabbir R.N., Hussain R.A., Anwar I., Aurangzaib M. Understanding brassinosteroid-regulated mechanisms to improve stress tolerance in plants: A critical review. Environ. Sci. Pollut. Res. Int. 2017;24:15959–15975. doi: 10.1007/s11356-017-9163-6. - DOI - PubMed
Publication types
MeSH terms
Substances
Grants and funding
LinkOut - more resources
Full Text Sources