Human ZKSCAN3 and Drosophila M1BP are functionally homologous transcription factors in autophagy regulation
- PMID: 32541927
- PMCID: PMC7296029
- DOI: 10.1038/s41598-020-66377-z
Human ZKSCAN3 and Drosophila M1BP are functionally homologous transcription factors in autophagy regulation
Abstract
Autophagy is an essential cellular process that maintains homeostasis by recycling damaged organelles and nutrients during development and cellular stress. ZKSCAN3 is the sole identified master transcriptional repressor of autophagy in human cell lines. How ZKSCAN3 achieves autophagy repression at the mechanistic or organismal level however still remains to be elucidated. Furthermore, Zkscan3 knockout mice display no discernable autophagy-related phenotypes, suggesting that there may be substantial differences in the regulation of autophagy between normal tissues and tumor cell lines. Here, we demonstrate that vertebrate ZKSCAN3 and Drosophila M1BP are functionally homologous transcription factors in autophagy repression. Expression of ZKSCAN3 in Drosophila prevents premature autophagy onset due to loss of M1BP function and conversely, M1BP expression in human cells can prevent starvation-induced autophagy due to loss of nuclear ZKSCAN3 function. In Drosophila ZKSCAN3 binds genome-wide to sequences targeted by M1BP and transcriptionally regulates the majority of M1BP-controlled genes, demonstrating the evolutionary conservation of the transcriptional repression of autophagy. This study thus allows the potential for transitioning the mechanisms, gene targets and plethora metabolic processes controlled by M1BP onto ZKSCAN3 and opens up Drosophila as a tool in studying the function of ZKSCAN3 in autophagy and tumourigenesis.
Conflict of interest statement
The authors declare no competing interests.
Figures
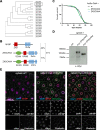
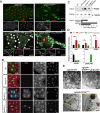
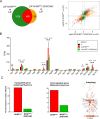
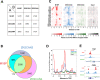
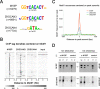
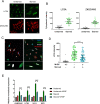
Similar articles
-
Transcriptional pausing factor M1BP regulates cellular homeostasis by suppressing autophagy and apoptosis in Drosophila eye.Autophagy Rep. 2023;2(1):2252307. doi: 10.1080/27694127.2023.2252307. Epub 2023 Sep 5. Autophagy Rep. 2023. PMID: 37746026 Free PMC article.
-
ZKSCAN3 is a master transcriptional repressor of autophagy.Mol Cell. 2013 Apr 11;50(1):16-28. doi: 10.1016/j.molcel.2013.01.024. Epub 2013 Feb 21. Mol Cell. 2013. PMID: 23434374 Free PMC article.
-
M1BP cooperates with CP190 to activate transcription at TAD borders and promote chromatin insulator activity.Nat Commun. 2021 Jul 7;12(1):4170. doi: 10.1038/s41467-021-24407-y. Nat Commun. 2021. PMID: 34234130 Free PMC article.
-
The DRE/DREF transcriptional regulatory system: a master key for cell proliferation.Biochim Biophys Acta. 2008 Feb;1779(2):81-9. doi: 10.1016/j.bbagrm.2007.11.011. Epub 2007 Dec 4. Biochim Biophys Acta. 2008. PMID: 18155677 Review.
-
The return of the nucleus: transcriptional and epigenetic control of autophagy.Nat Rev Mol Cell Biol. 2014 Jan;15(1):65-74. doi: 10.1038/nrm3716. Epub 2013 Dec 11. Nat Rev Mol Cell Biol. 2014. PMID: 24326622 Review.
Cited by
-
An RNA-interference screen in Drosophila to identify ZAD-containing C2H2 zinc finger genes that function in female germ cells.G3 (Bethesda). 2021 Jan 18;11(1):jkaa016. doi: 10.1093/g3journal/jkaa016. G3 (Bethesda). 2021. PMID: 33561227 Free PMC article.
-
Association between autophagy and acute pancreatitis.Front Genet. 2023 Jan 30;14:998035. doi: 10.3389/fgene.2023.998035. eCollection 2023. Front Genet. 2023. PMID: 36793898 Free PMC article. Review.
-
The Biological Roles of ZKSCAN3 (ZNF306) in the Hallmarks of Cancer: From Mechanisms to Therapeutics.Int J Mol Sci. 2024 Oct 27;25(21):11532. doi: 10.3390/ijms252111532. Int J Mol Sci. 2024. PMID: 39519085 Free PMC article. Review.
-
Overexpression of ZNF460 predicts worse survival and promotes metastasis through JAK2/STAT3 signaling pathway in patient with colon cancer.J Cancer. 2021 Apr 2;12(11):3198-3208. doi: 10.7150/jca.55079. eCollection 2021. J Cancer. 2021. PMID: 33976729 Free PMC article.
-
Transcriptional pausing factor M1BP regulates cellular homeostasis by suppressing autophagy and apoptosis in Drosophila eye.Autophagy Rep. 2023;2(1):2252307. doi: 10.1080/27694127.2023.2252307. Epub 2023 Sep 5. Autophagy Rep. 2023. PMID: 37746026 Free PMC article.
References
Publication types
MeSH terms
Substances
LinkOut - more resources
Full Text Sources
Molecular Biology Databases