Structures of human pannexin 1 reveal ion pathways and mechanism of gating
- PMID: 32494015
- PMCID: PMC7814660
- DOI: 10.1038/s41586-020-2357-y
Structures of human pannexin 1 reveal ion pathways and mechanism of gating
Abstract
Pannexin 1 (PANX1) is an ATP-permeable channel with critical roles in a variety of physiological functions such as blood pressure regulation1, apoptotic cell clearance2 and human oocyte development3. Here we present several structures of human PANX1 in a heptameric assembly at resolutions of up to 2.8 angström, including an apo state, a caspase-7-cleaved state and a carbenoxolone-bound state. We reveal a gating mechanism that involves two ion-conducting pathways. Under normal cellular conditions, the intracellular entry of the wide main pore is physically plugged by the C-terminal tail. Small anions are conducted through narrow tunnels in the intracellular domain. These tunnels connect to the main pore and are gated by a long linker between the N-terminal helix and the first transmembrane helix. During apoptosis, the C-terminal tail is cleaved by caspase, allowing the release of ATP through the main pore. We identified a carbenoxolone-binding site embraced by W74 in the extracellular entrance and a role for carbenoxolone as a channel blocker. We identified a gap-junction-like structure using a glycosylation-deficient mutant, N255A. Our studies provide a solid foundation for understanding the molecular mechanisms underlying the channel gating and inhibition of PANX1 and related large-pore channels.
Figures
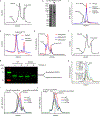
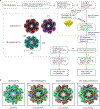
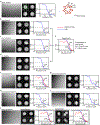
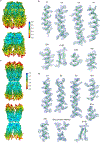
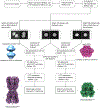
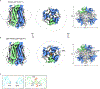
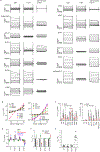
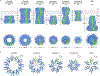
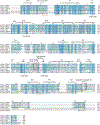
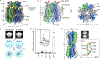
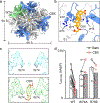
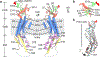
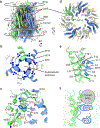
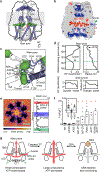
Similar articles
-
Depressing time: Waiting, melancholia, and the psychoanalytic practice of care.In: Kirtsoglou E, Simpson B, editors. The Time of Anthropology: Studies of Contemporary Chronopolitics. Abingdon: Routledge; 2020. Chapter 5. In: Kirtsoglou E, Simpson B, editors. The Time of Anthropology: Studies of Contemporary Chronopolitics. Abingdon: Routledge; 2020. Chapter 5. PMID: 36137063 Free Books & Documents. Review.
-
Dynamic Field Theory of Executive Function: Identifying Early Neurocognitive Markers.Monogr Soc Res Child Dev. 2024 Dec;89(3):7-109. doi: 10.1111/mono.12478. Monogr Soc Res Child Dev. 2024. PMID: 39628288 Free PMC article.
-
The C-terminal activating domain promotes pannexin 1 channel opening.Proc Natl Acad Sci U S A. 2024 Dec 17;121(51):e2411898121. doi: 10.1073/pnas.2411898121. Epub 2024 Dec 13. Proc Natl Acad Sci U S A. 2024. PMID: 39671183
-
Comparison of Two Modern Survival Prediction Tools, SORG-MLA and METSSS, in Patients With Symptomatic Long-bone Metastases Who Underwent Local Treatment With Surgery Followed by Radiotherapy and With Radiotherapy Alone.Clin Orthop Relat Res. 2024 Dec 1;482(12):2193-2208. doi: 10.1097/CORR.0000000000003185. Epub 2024 Jul 23. Clin Orthop Relat Res. 2024. PMID: 39051924
-
Platelet-rich therapies for musculoskeletal soft tissue injuries.Cochrane Database Syst Rev. 2014 Apr 29;2014(4):CD010071. doi: 10.1002/14651858.CD010071.pub3. Cochrane Database Syst Rev. 2014. PMID: 24782334 Free PMC article. Review.
Cited by
-
Amount of Pannexin 1 in Smooth Muscle Cells Regulates Sympathetic Nerve-Induced Vasoconstriction.Hypertension. 2023 Feb;80(2):416-425. doi: 10.1161/HYPERTENSIONAHA.122.20280. Epub 2022 Nov 30. Hypertension. 2023. PMID: 36448464 Free PMC article.
-
Age-Dependent Activation of Pannexin1 Function Contributes to the Development of Epileptogenesis in Autosomal Dominant Sleep-related Hypermotor Epilepsy Model Rats.Int J Mol Sci. 2024 Jan 28;25(3):1619. doi: 10.3390/ijms25031619. Int J Mol Sci. 2024. PMID: 38338895 Free PMC article.
-
Structure of the full-length human Pannexin1 channel and insights into its role in pyroptosis.Cell Discov. 2021 May 4;7(1):30. doi: 10.1038/s41421-021-00259-0. Cell Discov. 2021. PMID: 33947837 Free PMC article.
-
Cryo-EM structure of the heptameric calcium homeostasis modulator 1 channel.J Biol Chem. 2022 May;298(5):101838. doi: 10.1016/j.jbc.2022.101838. Epub 2022 Mar 24. J Biol Chem. 2022. PMID: 35339491 Free PMC article.
-
Structural and functional analysis of human pannexin 2 channel.Nat Commun. 2023 Mar 27;14(1):1712. doi: 10.1038/s41467-023-37413-z. Nat Commun. 2023. PMID: 36973289 Free PMC article.
References
Main text references
Additional References
-
- Zhou Q et al. Target protease specificity of the viral serpin CrmA. Analysis of five caspases. J. Biol. Chem 272, 7797–7800 (1997). - PubMed
-
- Denault J-B & Salvesen GS Expression, purification, and characterization of caspases. Curr. Protoc. protein Sci Chapter 21, Unit 21.13 (2003). - PubMed
-
- Mastronarde DN Automated electron microscope tomography using robust prediction of specimen movements. J. Struct. Biol 152, 36–51 (2005). - PubMed
Publication types
MeSH terms
Substances
Grants and funding
LinkOut - more resources
Full Text Sources
Molecular Biology Databases
Research Materials
Miscellaneous