TiPARP forms nuclear condensates to degrade HIF-1α and suppress tumorigenesis
- PMID: 32482854
- PMCID: PMC7306777
- DOI: 10.1073/pnas.1921815117
TiPARP forms nuclear condensates to degrade HIF-1α and suppress tumorigenesis
Abstract
Precisely controlling the activation of transcription factors is crucial for physiology. After a transcription factor is activated and carries out its transcriptional activity, it also needs to be properly deactivated. Here, we report a deactivation mechanism of HIF-1 and several other oncogenic transcription factors. HIF-1 promotes the transcription of an ADP ribosyltransferase, TiPARP, which serves to deactivate HIF-1. Mechanistically, TiPARP forms distinct nuclear condensates or nuclear bodies in an ADP ribosylation-dependent manner. The TiPARP nuclear bodies recruit both HIF-1α and an E3 ubiquitin ligase HUWE1, which promotes the ubiquitination and degradation of HIF-1α. Similarly, TiPARP promotes the degradation of c-Myc and estrogen receptor. By suppressing HIF-1α and other oncogenic transcription factors, TiPARP exerts strong antitumor effects both in cell culture and in mouse xenograft models. Our work reveals TiPARP as a negative-feedback regulator for multiple oncogenic transcription factors, provides insights into the functions of protein ADP-ribosylation, and suggests activating TiPARP as an anticancer strategy.
Keywords: ADP-ribosylation; HIF-1; TiPARP; nuclear condensates; ubiquitination.
Copyright © 2020 the Author(s). Published by PNAS.
Conflict of interest statement
The authors declare no competing interest.
Figures
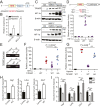
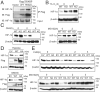
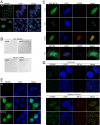
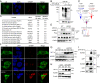
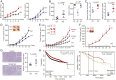
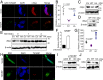
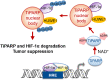
Similar articles
-
Roles of the ubiquitin ligase CUL4B and ADP-ribosyltransferase TiPARP in TCDD-induced nuclear export and proteasomal degradation of the transcription factor AHR.J Biol Chem. 2021 Aug;297(2):100886. doi: 10.1016/j.jbc.2021.100886. Epub 2021 Jun 16. J Biol Chem. 2021. PMID: 34146543 Free PMC article.
-
TCDD-inducible poly-ADP-ribose polymerase (TIPARP/PARP7) mono-ADP-ribosylates and co-activates liver X receptors.Biochem J. 2016 Apr 1;473(7):899-910. doi: 10.1042/BJ20151077. Epub 2016 Jan 26. Biochem J. 2016. PMID: 26814197
-
Characterization of TCDD-inducible poly-ADP-ribose polymerase (TIPARP/ARTD14) catalytic activity.Biochem J. 2018 Dec 11;475(23):3827-3846. doi: 10.1042/BCJ20180347. Biochem J. 2018. PMID: 30373764 Free PMC article.
-
RACK1 vs. HSP90: competition for HIF-1 alpha degradation vs. stabilization.Cell Cycle. 2007 Mar 15;6(6):656-9. doi: 10.4161/cc.6.6.3981. Epub 2007 Mar 7. Cell Cycle. 2007. PMID: 17361105 Review.
-
The functional interplay between the HIF pathway and the ubiquitin system - more than a one-way road.Exp Cell Res. 2017 Jul 15;356(2):152-159. doi: 10.1016/j.yexcr.2017.03.027. Epub 2017 Mar 15. Exp Cell Res. 2017. PMID: 28315321 Review.
Cited by
-
Aryl Hydrocarbon Receptor Mechanisms Affecting Chronic Kidney Disease.Front Pharmacol. 2022 Feb 14;13:782199. doi: 10.3389/fphar.2022.782199. eCollection 2022. Front Pharmacol. 2022. PMID: 35237156 Free PMC article. Review.
-
Regulation of Biomolecular Condensates by Poly(ADP-ribose).Chem Rev. 2023 Jul 26;123(14):9065-9093. doi: 10.1021/acs.chemrev.2c00851. Epub 2023 Apr 28. Chem Rev. 2023. PMID: 37115110 Free PMC article. Review.
-
Identification of PARP-7 substrates reveals a role for MARylation in microtubule control in ovarian cancer cells.Elife. 2021 Jan 21;10:e60481. doi: 10.7554/eLife.60481. Elife. 2021. PMID: 33475085 Free PMC article.
-
PARP1 condensates differentially partition DNA repair proteins and enhance DNA ligation.EMBO Rep. 2024 Dec;25(12):5635-5666. doi: 10.1038/s44319-024-00285-5. Epub 2024 Nov 4. EMBO Rep. 2024. PMID: 39496836 Free PMC article.
-
Role of sex steroids in colorectal cancer: pathomechanisms and medical applications.Am J Cancer Res. 2024 Jul 15;14(7):3200-3221. doi: 10.62347/OEBS6893. eCollection 2024. Am J Cancer Res. 2024. PMID: 39113870 Free PMC article. Review.
References
-
- Gibson B. A., Kraus W. L., New insights into the molecular and cellular functions of poly(ADP-ribose) and PARPs. Nat. Rev. Mol. Cell Biol. 13, 411–424 (2012). - PubMed
-
- Hottiger M. O., Hassa P. O., Lüscher B., Schüler H., Koch-Nolte F., Toward a unified nomenclature for mammalian ADP-ribosyltransferases. Trends Biochem. Sci. 35, 208–219 (2010). - PubMed
-
- Corda D., Di Girolamo M., Mono-ADP-ribosylation: A tool for modulating immune response and cell signaling. Sci. STKE 2002, pe53 (2002). - PubMed
Publication types
MeSH terms
Substances
Grants and funding
LinkOut - more resources
Full Text Sources
Miscellaneous