Sperm Differentiation: The Role of Trafficking of Proteins
- PMID: 32456358
- PMCID: PMC7279445
- DOI: 10.3390/ijms21103702
Sperm Differentiation: The Role of Trafficking of Proteins
Abstract
Sperm differentiation encompasses a complex sequence of morphological changes that takes place in the seminiferous epithelium. In this process, haploid round spermatids undergo substantial structural and functional alterations, resulting in highly polarized sperm. Hallmark changes during the differentiation process include the formation of new organelles, chromatin condensation and nuclear shaping, elimination of residual cytoplasm, and assembly of the sperm flagella. To achieve these transformations, spermatids have unique mechanisms for protein trafficking that operate in a coordinated fashion. Microtubules and filaments of actin are the main tracks used to facilitate the transport mechanisms, assisted by motor and non-motor proteins, for delivery of vesicular and non-vesicular cargos to specific sites. This review integrates recent findings regarding the role of protein trafficking in sperm differentiation. Although a complete characterization of the interactome of proteins involved in these temporal and spatial processes is not yet known, we propose a model based on the current literature as a framework for future investigations.
Keywords: acrosome; manchette; protein trafficking; sperm differentiation.
Conflict of interest statement
The authors declare no conflicts of interest.
Figures
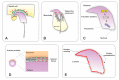
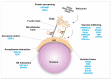
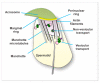
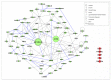
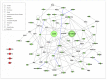
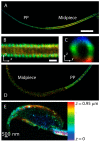
Similar articles
-
Haploid male germ cells-the Grand Central Station of protein transport.Hum Reprod Update. 2020 Jun 18;26(4):474-500. doi: 10.1093/humupd/dmaa004. Hum Reprod Update. 2020. PMID: 32318721 Review.
-
Formation and function of the manchette and flagellum during spermatogenesis.Reproduction. 2016 Apr;151(4):R43-54. doi: 10.1530/REP-15-0310. Epub 2016 Jan 20. Reproduction. 2016. PMID: 26792866 Review.
-
Manchette-acrosome disorders during spermiogenesis and low efficiency of seminiferous tubules in hypercholesterolemic rabbit model.PLoS One. 2017 Feb 27;12(2):e0172994. doi: 10.1371/journal.pone.0172994. eCollection 2017. PLoS One. 2017. PMID: 28241054 Free PMC article.
-
The postacrosomal assembly of sperm head protein, PAWP, is independent of acrosome formation and dependent on microtubular manchette transport.Dev Biol. 2007 Dec 15;312(2):471-83. doi: 10.1016/j.ydbio.2007.08.051. Epub 2007 Sep 7. Dev Biol. 2007. PMID: 17988661
-
Spermatogenesis revisited. III. The course of spermatogenesis in a male-sterile pink-eyed mutant type in the mouse.Cell Tissue Res. 1977 May 16;180(2):173-86. doi: 10.1007/BF00231950. Cell Tissue Res. 1977. PMID: 872192
Cited by
-
Map-1a regulates Sertoli cell BTB dynamics through the cytoskeletal organization of microtubule and F-actin.Reprod Biol Endocrinol. 2024 Apr 3;22(1):36. doi: 10.1186/s12958-024-01204-y. Reprod Biol Endocrinol. 2024. PMID: 38570783 Free PMC article.
-
Differentiation Disorders of Chara vulgaris Spermatids following Treatment with Propyzamide.Cells. 2023 Apr 27;12(9):1268. doi: 10.3390/cells12091268. Cells. 2023. PMID: 37174667 Free PMC article.
-
Distinct patterns of gene expression during regeneration and asexual reproduction in the annelid Pristina leidyi.J Exp Zool B Mol Dev Evol. 2022 Nov;338(7):405-420. doi: 10.1002/jez.b.23143. Epub 2022 May 23. J Exp Zool B Mol Dev Evol. 2022. PMID: 35604322 Free PMC article.
-
ADAD1 is required for normal translation of nuclear pore and transport protein transcripts in spermatids of Mus musculus†.Biol Reprod. 2023 Sep 12;109(3):340-355. doi: 10.1093/biolre/ioad069. Biol Reprod. 2023. PMID: 37399121 Free PMC article.
-
BAG5 regulates HSPA8-mediated protein folding required for sperm head-tail coupling apparatus assembly.EMBO Rep. 2024 Apr;25(4):2045-2070. doi: 10.1038/s44319-024-00112-x. Epub 2024 Mar 7. EMBO Rep. 2024. PMID: 38454159 Free PMC article.
References
-
- Russell L.D., Ettlin R.A., Sinha Hikim A.P., Clegg E.D. Cache River Press; St. Louis, MO, USA: 1990. Histological and Histopathological Evaluation of the Testis.
-
- Auger J. Spermatozoa and Sperm Structure. In: Skinner M., editor. Encyclopedia of Reproduction. 2nd ed. Academic Press; Amsterdam, The Netherlands: 2018. pp. 62–67.
-
- Cohen J. Reproduction. Butterworth & Co.; London, UK: 1977.
-
- Birkhead T.R., Hosken D.J., Pitnick S.B.T. Biology of Spermatozoa Sperm Biology. Academic Press; Amsterdam, The Netherlands: 2009.
-
- Temple-Smith P., Ravichandran A., Horta F. Sperm: Comparative Vertebrate. In: Skinner M., editor. Encyclopedia of Reproduction. 2nd ed. Academic Press; Amsterdam, The Netherlands: 2018. pp. 210–220.
Publication types
MeSH terms
Substances
Grants and funding
- CGL2016-80577-P/Spanish Ministry of Science, Innovation and Universities/International
- 2019AEP165/Consejo Superior de Investigaciones Científicas/International
- U54HD3449/NH/NIH HHS/United States
- R01HD083323/NH/NIH HHS/United States
- UL1TR000058/VCU's, CTSA ( from the National Center for Advancing Translational Science) and CCTR Endowment Fund of Virginia Commonwealth University/International
LinkOut - more resources
Full Text Sources