DCyFIR: a high-throughput CRISPR platform for multiplexed G protein-coupled receptor profiling and ligand discovery
- PMID: 32434907
- PMCID: PMC7293659
- DOI: 10.1073/pnas.2000430117
DCyFIR: a high-throughput CRISPR platform for multiplexed G protein-coupled receptor profiling and ligand discovery
Abstract
More than 800 G protein-coupled receptors (GPCRs) comprise the largest class of membrane receptors in humans. While there is ample biological understanding and many approved drugs for prototypic GPCRs, most GPCRs still lack well-defined biological ligands and drugs. Here, we report our efforts to tap the potential of understudied GPCRs by developing yeast-based technologies for high-throughput clustered regularly interspaced short palindromic repeats (CRISPR) engineering and GPCR ligand discovery. We refer to these technologies collectively as Dynamic Cyan Induction by Functional Integrated Receptors, or DCyFIR. A major advantage of DCyFIR is that GPCRs and other assay components are CRISPR-integrated directly into the yeast genome, making it possible to decode ligand specificity by profiling mixtures of GPCR-barcoded yeast strains in a single tube. To demonstrate the capabilities of DCyFIR, we engineered a yeast strain library of 30 human GPCRs and their 300 possible GPCR-Gα coupling combinations. Profiling of these 300 strains, using parallel (DCyFIRscreen) and multiplex (DCyFIRplex) DCyFIR modes, recapitulated known GPCR agonism with 100% accuracy, and identified unexpected interactions for the receptors ADRA2B, HCAR3, MTNR1A, S1PR1, and S1PR2. To demonstrate DCyFIR scalability, we profiled a library of 320 human metabolites and discovered several GPCR-metabolite interactions. Remarkably, many of these findings pertained to understudied pharmacologically dark receptors GPR4, GPR65, GPR68, and HCAR3. Experiments on select receptors in mammalian cells confirmed our yeast-based observations, including our discovery that kynurenic acid activates HCAR3 in addition to GPR35, its known receptor. Taken together, these findings demonstrate the power of DCyFIR for identifying ligand interactions with prototypic and understudied GPCRs.
Keywords: DCyFIR; G protein-coupled receptors; multiplex; pharmacologically dark GPCR; yeast.
Conflict of interest statement
The authors declare no competing interest.
Figures
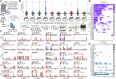
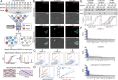
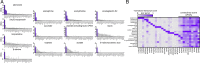
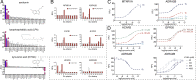
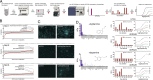
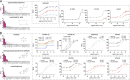

Similar articles
-
CRISPR-addressable yeast strains with applications in human G protein-coupled receptor profiling and synthetic biology.J Biol Chem. 2020 Jun 12;295(24):8262-8271. doi: 10.1074/jbc.RA120.013066. Epub 2020 May 1. J Biol Chem. 2020. PMID: 32358068 Free PMC article.
-
The evolution and mechanism of GPCR proton sensing.J Biol Chem. 2021 Jan-Jun;296:100167. doi: 10.1074/jbc.RA120.016352. Epub 2020 Dec 13. J Biol Chem. 2021. PMID: 33478938 Free PMC article.
-
Human G protein-coupled receptor studies in Saccharomyces cerevisiae.Biochem Pharmacol. 2016 Aug 15;114:103-15. doi: 10.1016/j.bcp.2016.02.010. Epub 2016 Feb 23. Biochem Pharmacol. 2016. PMID: 26920251 Review.
-
GPCR profiling: from hits to leads and from genotype to phenotype.Drug Discov Today Technol. 2015 Nov;18:30-7. doi: 10.1016/j.ddtec.2015.10.005. Epub 2015 Nov 6. Drug Discov Today Technol. 2015. PMID: 26723890 Review.
-
Engineering cell sensing and responses using a GPCR-coupled CRISPR-Cas system.Nat Commun. 2017 Dec 20;8(1):2212. doi: 10.1038/s41467-017-02075-1. Nat Commun. 2017. PMID: 29263378 Free PMC article.
Cited by
-
Rational engineering approaches for establishing insect olfaction reporters in yeast.Biotechnol Notes. 2023 Nov 17;4:90-99. doi: 10.1016/j.biotno.2023.11.002. eCollection 2023. Biotechnol Notes. 2023. PMID: 39416924 Free PMC article.
-
Engineered cell differentiation and sexual reproduction in probiotic and mating yeasts.Nat Commun. 2022 Oct 19;13(1):6201. doi: 10.1038/s41467-022-33961-y. Nat Commun. 2022. PMID: 36261657 Free PMC article.
-
Olfactory Receptors as an Emerging Chemical Sensing Scaffold.Biochemistry. 2023 Jan 17;62(2):187-195. doi: 10.1021/acs.biochem.2c00486. Epub 2022 Nov 1. Biochemistry. 2023. PMID: 36318941 Free PMC article. Review.
-
Peptide-Dependent Growth in Yeast via Fine-Tuned Peptide/GPCR-Activated Essential Gene Expression.Biochemistry. 2022 Feb 1;61(3):150-159. doi: 10.1021/acs.biochem.1c00661. Epub 2022 Jan 13. Biochemistry. 2022. PMID: 35023728 Free PMC article.
-
Orphan G protein-coupled receptors: the ongoing search for a home.Front Pharmacol. 2024 Feb 29;15:1349097. doi: 10.3389/fphar.2024.1349097. eCollection 2024. Front Pharmacol. 2024. PMID: 38495099 Free PMC article. Review.
References
Publication types
MeSH terms
Substances
Grants and funding
LinkOut - more resources
Full Text Sources
Other Literature Sources
Molecular Biology Databases
Miscellaneous