Spike Activity Regulates Vesicle Filling at a Glutamatergic Synapse
- PMID: 32430294
- PMCID: PMC7314407
- DOI: 10.1523/JNEUROSCI.2945-19.2020
Spike Activity Regulates Vesicle Filling at a Glutamatergic Synapse
Abstract
Synaptic vesicles need to be recycled and refilled rapidly to maintain high-frequency synaptic transmission. However, little is known about the control of neurotransmitter transport into synaptic vesicles, which determines the contents of synaptic vesicles and the strength of synaptic transmission. Here, we report that Na+ substantially accumulated in the calyx of Held terminals of juvenile mice of either sex during high-frequency spiking. The activity-induced elevation of cytosolic Na+ activated vesicular Na+/H+ exchanger, facilitated glutamate loading into synaptic vesicles, and increased quantal size of asynchronous released vesicles but did not affect the vesicle pool size or release probability. Consequently, presynaptic Na+ increased the EPSCs and was required to maintain the reliable high-frequency signal transmission from the presynaptic calyces to the postsynaptic medial nucleus of the trapezoid body (MNTB) neurons. Blocking Na+/H+ exchange activity decreased the postsynaptic current and caused failures in postsynaptic firing. Therefore, during high-frequency synaptic transmission, when large amounts of glutamate are released, Na+ accumulated in the terminals, activated vesicular Na+/H+ exchanger, and regulated glutamate loading as a function of the level of vesicle release.SIGNIFICANCE STATEMENT Auditory information is encoded by action potentials (APs) phase-locked to sound frequency at high rates. A large number of synaptic vesicles are released during high-frequency synaptic transmission; accordingly, synaptic vesicles need to be recycled and refilled rapidly. We have recently found that a Na+/H+ exchanger expressed on synaptic vesicles promotes vesicle filling with glutamate. Here, we showed that when a large number of synaptic vesicles are released during high-frequency synaptic transmission, Na+ accumulates in axon terminals and facilitates glutamate uptake into synaptic vesicles. Na+ thus accelerates vesicle replenishment and sustains reliable synaptic transmission.
Keywords: axon terminal; calyx of Held; glutamate; spiking; synaptic vesicle; vesicular glutamate transport.
Copyright © 2020 the authors.
Figures
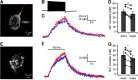
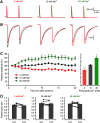
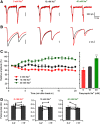
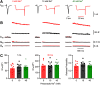
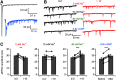
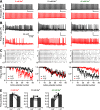
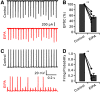
Similar articles
-
Activity and Cytosolic Na+ Regulate Synaptic Vesicle Endocytosis.J Neurosci. 2020 Aug 5;40(32):6112-6120. doi: 10.1523/JNEUROSCI.0119-20.2020. Epub 2020 Jun 30. J Neurosci. 2020. PMID: 32605936 Free PMC article.
-
Presynaptic HCN channels regulate vesicular glutamate transport.Neuron. 2014 Oct 22;84(2):340-6. doi: 10.1016/j.neuron.2014.08.046. Epub 2014 Sep 25. Neuron. 2014. PMID: 25263752 Free PMC article.
-
Impact of vesicular glutamate leakage on synaptic transmission at the calyx of Held.J Physiol. 2017 Feb 15;595(4):1263-1271. doi: 10.1113/JP273467. Epub 2016 Nov 29. J Physiol. 2017. PMID: 27801501 Free PMC article.
-
Synaptic vesicle protein trafficking at the glutamate synapse.Neuroscience. 2009 Jan 12;158(1):189-203. doi: 10.1016/j.neuroscience.2008.03.029. Epub 2008 Mar 22. Neuroscience. 2009. PMID: 18472224 Free PMC article. Review.
-
Vesicle pools and short-term synaptic depression: lessons from a large synapse.Trends Neurosci. 2002 Apr;25(4):206-12. doi: 10.1016/s0166-2236(02)02139-2. Trends Neurosci. 2002. PMID: 11998689 Review.
Cited by
-
SCAMP5 plays a critical role in axonal trafficking and synaptic localization of NHE6 to adjust quantal size at glutamatergic synapses.Proc Natl Acad Sci U S A. 2021 Jan 12;118(2):e2011371118. doi: 10.1073/pnas.2011371118. Proc Natl Acad Sci U S A. 2021. PMID: 33372133 Free PMC article.
-
SCAMP5 mediates activity-dependent enhancement of NHE6 recruitment to synaptic vesicles during synaptic plasticity.Mol Brain. 2021 Mar 4;14(1):47. doi: 10.1186/s13041-021-00763-0. Mol Brain. 2021. PMID: 33663553 Free PMC article.
-
Activity and Cytosolic Na+ Regulate Synaptic Vesicle Endocytosis.J Neurosci. 2020 Aug 5;40(32):6112-6120. doi: 10.1523/JNEUROSCI.0119-20.2020. Epub 2020 Jun 30. J Neurosci. 2020. PMID: 32605936 Free PMC article.
-
A mean-field model of glutamate and GABA synaptic dynamics for functional MRS.Neuroimage. 2023 Feb 1;266:119813. doi: 10.1016/j.neuroimage.2022.119813. Epub 2022 Dec 14. Neuroimage. 2023. PMID: 36528313 Free PMC article.
-
Alkalinization of the Synaptic Cleft during Excitatory Neurotransmission.J Neurosci. 2020 Aug 12;40(33):6267-6269. doi: 10.1523/JNEUROSCI.0914-20.2020. J Neurosci. 2020. PMID: 32801127 Free PMC article. No abstract available.
References
Publication types
MeSH terms
Substances
Grants and funding
LinkOut - more resources
Full Text Sources
Other Literature Sources
Miscellaneous