Synergistic Effect of Cell-Derived Extracellular Matrices and Topography on Osteogenesis of Mesenchymal Stem Cells
- PMID: 32423202
- PMCID: PMC7291345
- DOI: 10.1021/acsami.0c05012
Synergistic Effect of Cell-Derived Extracellular Matrices and Topography on Osteogenesis of Mesenchymal Stem Cells
Abstract
Cell-derived matrices (CDMs) are an interesting alternative to conventional sources of extracellular matrices (ECMs) as CDMs mimic the natural ECM composition better and are therefore attractive as a scaffolding material for regulating the functions of stem cells. Previous research on stem cell differentiation has demonstrated that both surface topography and CDMs have a significant influence. However, not much focus has been devoted to elucidating possible synergistic effects of CDMs and topography on osteogenic differentiation of human bone marrow-derived mesenchymal stem cells (hBM-MSCs). In this study, polydimethylsiloxane (PDMS)-based anisotropic topographies (wrinkles) with various topography dimensions were prepared and subsequently combined with native ECMs produced by human fibroblasts that remained on the surface topography after decellularization. The synergistic effect of CDMs combined with topography on osteogenic differentiation of hBM-MSCs was investigated. The results showed that substrates with specific topography dimensions, coated with aligned CDMs, dramatically enhanced the capacity of osteogenesis as investigated using immunofluorescence staining for identifying osteopontin (OPN) and mineralization. Furthermore, the hBM-MSCs on the substrates decorated with CDMs exhibited a higher percentage of (Yes-associated protein) YAP inside the nucleus, stronger cell contractility, and greater formation of focal adhesions, illustrating that enhanced osteogenesis is partly mediated by cellular tension and mechanotransduction following the YAP pathway. Taken together, our findings highlight the importance of ECMs mediating the osteogenic differentiation of stem cells, and the combination of CDMs and topography will be a powerful approach for material-driven osteogenesis.
Keywords: extracellular matrix; mechanotransduction; mesenchymal stem cells; osteogenic differentiation; topography.
Conflict of interest statement
The authors declare the following competing financial interest(s): P.v.R. also is co-founder, scientific advisor, and share-holder of BiomACS BV, a biomedical oriented screening company. The authors declare no other competing interests.
Figures
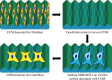
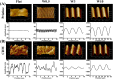
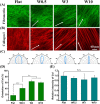
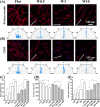
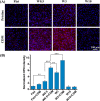
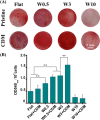
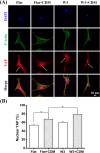
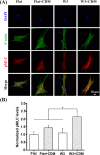
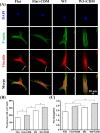
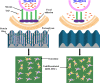
Similar articles
-
Extracellular matrix type modulates mechanotransduction of stem cells.Acta Biomater. 2019 Sep 15;96:310-320. doi: 10.1016/j.actbio.2019.06.048. Epub 2019 Jun 28. Acta Biomater. 2019. PMID: 31255664 Free PMC article.
-
Type I collagen deposition via osteoinduction ameliorates YAP/TAZ activity in 3D floating culture clumps of mesenchymal stem cell/extracellular matrix complexes.Stem Cell Res Ther. 2018 Dec 7;9(1):342. doi: 10.1186/s13287-018-1085-9. Stem Cell Res Ther. 2018. PMID: 30526677 Free PMC article.
-
Enhanced osteogenic differentiation of MC3T3-E1 cells on grid-topographic surface and evidence for involvement of YAP mediator.J Biomed Mater Res A. 2016 May;104(5):1143-52. doi: 10.1002/jbm.a.35648. Epub 2016 Feb 2. J Biomed Mater Res A. 2016. PMID: 26748630
-
Nanopattern-induced osteogenic differentiation of stem cells - A systematic review.Acta Biomater. 2016 Dec;46:3-14. doi: 10.1016/j.actbio.2016.09.031. Epub 2016 Sep 22. Acta Biomater. 2016. PMID: 27667018 Review.
-
Effects of nanofibers on mesenchymal stem cells: environmental factors affecting cell adhesion and osteogenic differentiation and their mechanisms.J Zhejiang Univ Sci B. 2020 Nov.;21(11):871-884. doi: 10.1631/jzus.B2000355. J Zhejiang Univ Sci B. 2020. PMID: 33150771 Free PMC article. Review.
Cited by
-
Cell-Derived Matrix: Production, Decellularization, and Application of Wound Repair.Stem Cells Int. 2024 May 29;2024:7398473. doi: 10.1155/2024/7398473. eCollection 2024. Stem Cells Int. 2024. PMID: 38882595 Free PMC article. Review.
-
Self-agglomerated collagen patterns govern cell behaviour.Sci Rep. 2021 Jan 15;11(1):1516. doi: 10.1038/s41598-021-81054-5. Sci Rep. 2021. PMID: 33452334 Free PMC article.
-
Sound innovations for biofabrication and tissue engineering.Microsyst Nanoeng. 2024 Nov 19;10(1):170. doi: 10.1038/s41378-024-00759-5. Microsyst Nanoeng. 2024. PMID: 39562793 Free PMC article. Review.
-
A Scalable Method to Fabricate 2D Hydrogel Substrates for Mechanobiology Studies with Independent Tuning of Adhesiveness and Stiffness.Methods Protoc. 2024 Sep 26;7(5):75. doi: 10.3390/mps7050075. Methods Protoc. 2024. PMID: 39452789 Free PMC article.
-
Decellularized Lung Extracellular Matrix Scaffold Promotes Human Embryonic Stem Cell Differentiation towards Alveolar Progenitors.Cell J. 2023 Jun 28;25(6):372-382. doi: 10.22074/cellj.2023.563370.1148. Cell J. 2023. PMID: 37434454 Free PMC article.
References
-
- Poudineh M.; Wang Z.; Labib M.; Ahmadi M.; Zhang L.; Das J.; Ahmed S. U.; Angers S.; Kelley S. O. Three-Dimensional Nanostructured Architectures Enable Efficient Neural Differentiation of Mesenchymal Stem Cells via Mechanotransduction. Nano Lett. 2018, 18, 7188–7193. 10.1021/acs.nanolett.8b03313. - DOI - PubMed
MeSH terms
Substances
LinkOut - more resources
Full Text Sources
Research Materials
Miscellaneous