The Emerging Role of the Mammalian Glycocalyx in Functional Membrane Organization and Immune System Regulation
- PMID: 32351961
- PMCID: PMC7174505
- DOI: 10.3389/fcell.2020.00253
The Emerging Role of the Mammalian Glycocalyx in Functional Membrane Organization and Immune System Regulation
Abstract
All cells in the human body are covered by a dense layer of sugars and the proteins and lipids to which they are attached, collectively termed the "glycocalyx." For decades, the organization of the glycocalyx and its interplay with the cellular state have remained enigmatic. This changed in recent years. Latest research has shown that the glycocalyx is an organelle of vital significance, actively involved in and functionally relevant for various cellular processes, that can be directly targeted in therapeutic contexts. This review gives a brief introduction into glycocalyx biology and describes the specific challenges glycocalyx research faces. Then, the traditional view of the role of the glycocalyx is discussed before several recent breakthroughs in glycocalyx research are surveyed. These results exemplify a currently unfolding bigger picture about the role of the glycocalyx as a fundamental cellular agent.
Keywords: KRAS; cancer; cancer immune therapy; glycocalyx; immune system; immunosynapse; membrane organization; siglecs.
Copyright © 2020 Möckl.
Figures
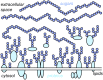
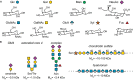
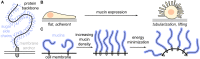
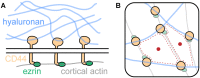
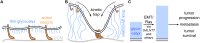
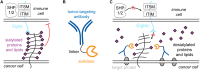
Similar articles
-
Setting the stage for universal pharmacological targeting of the glycocalyx.Curr Top Membr. 2023;91:61-88. doi: 10.1016/bs.ctm.2023.02.004. Epub 2023 Mar 10. Curr Top Membr. 2023. PMID: 37080681 Review.
-
Glycocalyx Curving the Membrane: Forces Emerging from the Cell Exterior.Annu Rev Cell Dev Biol. 2021 Oct 6;37:257-283. doi: 10.1146/annurev-cellbio-120219-054401. Annu Rev Cell Dev Biol. 2021. PMID: 34613816 Free PMC article. Review.
-
Biophysical modeling of membrane curvature generation and curvature sensing by the glycocalyx.bioRxiv [Preprint]. 2024 Sep 8:2024.09.07.611813. doi: 10.1101/2024.09.07.611813. bioRxiv. 2024. Update in: Proc Natl Acad Sci U S A. 2025 Feb 25;122(8):e2418357122. doi: 10.1073/pnas.2418357122. PMID: 39282261 Free PMC article. Updated. Preprint.
-
Sensing the neuronal glycocalyx by glial sialic acid binding immunoglobulin-like lectins.Neuroscience. 2014 Sep 5;275:113-24. doi: 10.1016/j.neuroscience.2014.05.061. Epub 2014 Jun 9. Neuroscience. 2014. PMID: 24924144 Review.
-
The role of the cell surface glycocalyx in drug delivery to and through the endothelium.Adv Drug Deliv Rev. 2022 May;184:114195. doi: 10.1016/j.addr.2022.114195. Epub 2022 Mar 12. Adv Drug Deliv Rev. 2022. PMID: 35292326 Review.
Cited by
-
Measuring the multifaceted roles of mucin-domain glycoproteins in cancer.Adv Cancer Res. 2023;157:83-121. doi: 10.1016/bs.acr.2022.09.001. Epub 2022 Oct 8. Adv Cancer Res. 2023. PMID: 36725114 Free PMC article. Review.
-
Mucin-mimetic glycan arrays integrating machine learning for analyzing receptor pattern recognition by influenza A viruses.Chem. 2021 Dec 9;7(12):3393-3411. doi: 10.1016/j.chempr.2021.09.015. Epub 2021 Oct 22. Chem. 2021. PMID: 34993358 Free PMC article.
-
Selective Quantification of Bacteria in Mixtures by Using Glycosylated Polypyrrole/Hydrogel Nanolayers.ACS Appl Mater Interfaces. 2024 Mar 20;16(11):14243-14251. doi: 10.1021/acsami.3c14387. Epub 2024 Mar 5. ACS Appl Mater Interfaces. 2024. PMID: 38442898 Free PMC article.
-
The unremarkable alveolar epithelial glycocalyx: a thorium dioxide-based electron microscopic comparison after heparinase or pneumolysin treatment.Histochem Cell Biol. 2023 Aug;160(2):83-96. doi: 10.1007/s00418-023-02211-7. Epub 2023 Jun 29. Histochem Cell Biol. 2023. PMID: 37386200 Free PMC article.
-
The Endothelial Glycocalyx: A Possible Therapeutic Target in Cardiovascular Disorders.Front Cardiovasc Med. 2022 May 13;9:897087. doi: 10.3389/fcvm.2022.897087. eCollection 2022. Front Cardiovasc Med. 2022. PMID: 35647072 Free PMC article. Review.
References
-
- Al-Nedawi K., Meehan B., Micallef J., Lhotak V., May L., Guha A., et al. (2008). Intercellular transfer of the oncogenic receptor EGFrvIII by microvesicles derived from tumour cells. Nat. Cell Biol. 10 619–U624. - PubMed
-
- Antonyak M. A., Li B., Boroughs L. K., Johnson J. L., Druso J. E., Bryant K. L., et al. (2011). Cancer cell-derived microvesicles induce transformation by transferring tissue transglutaminase and fibronectin to recipient cells. Proc. Natl. Acad. Sci. US A. 108 4852–4857. 10.1073/pnas.1017667108 - DOI - PMC - PubMed
Publication types
Grants and funding
LinkOut - more resources
Full Text Sources
Other Literature Sources
Miscellaneous