The Small GTPases Rab27b Regulates Mitochondrial Fatty Acid Oxidative Metabolism of Cardiac Mesenchymal Stem Cells
- PMID: 32351955
- PMCID: PMC7174509
- DOI: 10.3389/fcell.2020.00209
The Small GTPases Rab27b Regulates Mitochondrial Fatty Acid Oxidative Metabolism of Cardiac Mesenchymal Stem Cells
Abstract
Cardiac mesenchymal stem cells (C-MSCs) are endogenous cardiac stromal cells that play a crucial role in maintaining normal cardiac function. Rab27b is a member of the small GTPase Rab family that controls membrane trafficking and the secretion of exosomes. However, its role in regulating energy metabolism in C-MSC is unclear. In this study, we analyzed mitochondrial oxidative phosphorylation by quantifying cellular oxygen consumption rate (OCR) and quantified the extracellular acidification rate (ECAR) in C-MSC with/without Rab27b knockdown. Knockdown of Rab27b increased glycolysis, but significantly reduced mitochondrial oxidative phosphorylation (OXPHOS) with loss of mitochondrial membrane potential in C-MSC. Furthermore, knockdown of Rab27b reduced H3k4me3 expression in C-MSC and selectively decreased the expression of the essential genes involved in β-oxidation, tricarboxylic acid cycle (TCA), and electron transport chain (ETC). Taken together, our findings highlight a novel role for Rab27b in maintaining fatty acid oxidation in C-MSCs.
Keywords: Rab27b; cardiac mesenchymal stem cells; exosome; fatty acid oxidation; mitochondrial oxidative metabolism.
Copyright © 2020 Jin, Shen, Su, Cai, Liu, Weintraub and Tang.
Figures
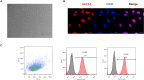
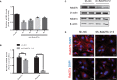
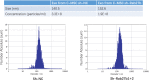
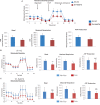
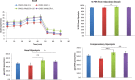
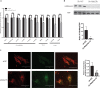
Similar articles
-
RNAase III-Type Enzyme Dicer Regulates Mitochondrial Fatty Acid Oxidative Metabolism in Cardiac Mesenchymal Stem Cells.Int J Mol Sci. 2019 Nov 7;20(22):5554. doi: 10.3390/ijms20225554. Int J Mol Sci. 2019. PMID: 31703292 Free PMC article.
-
Suxiao Jiuxin pill promotes exosome secretion from mouse cardiac mesenchymal stem cells in vitro.Acta Pharmacol Sin. 2018 Apr;39(4):569-578. doi: 10.1038/aps.2018.19. Epub 2018 Mar 15. Acta Pharmacol Sin. 2018. PMID: 29542682 Free PMC article.
-
Human multipotent mesenchymal stromal cells cytokine priming promotes RAB27B-regulated secretion of small extracellular vesicles with immunomodulatory cargo.Stem Cell Res Ther. 2020 Dec 14;11(1):539. doi: 10.1186/s13287-020-02050-6. Stem Cell Res Ther. 2020. PMID: 33317598 Free PMC article.
-
Mitochondrial Transfer and Regulators of Mesenchymal Stromal Cell Function and Therapeutic Efficacy.Front Cell Dev Biol. 2020 Dec 7;8:603292. doi: 10.3389/fcell.2020.603292. eCollection 2020. Front Cell Dev Biol. 2020. PMID: 33365311 Free PMC article. Review.
-
Musculoskeletal Progenitor/Stromal Cell-Derived Mitochondria Modulate Cell Differentiation and Therapeutical Function.Front Immunol. 2021 Mar 8;12:606781. doi: 10.3389/fimmu.2021.606781. eCollection 2021. Front Immunol. 2021. PMID: 33763061 Free PMC article. Review.
Cited by
-
Transcriptome Analysis on Key Metabolic Pathways in Rhodotorula mucilaginosa Under Pb(II) Stress.Appl Environ Microbiol. 2022 Apr 12;88(7):e0221521. doi: 10.1128/aem.02215-21. Epub 2022 Mar 21. Appl Environ Microbiol. 2022. PMID: 35311507 Free PMC article.
-
Renal cancer secretome induces migration of mesenchymal stromal cells.Stem Cell Res Ther. 2023 Aug 10;14(1):200. doi: 10.1186/s13287-023-03430-4. Stem Cell Res Ther. 2023. PMID: 37563650 Free PMC article.
-
The role and mechanism of mitochondrial functions and energy metabolism in the function regulation of the mesenchymal stem cells.Stem Cell Res Ther. 2021 Feb 17;12(1):140. doi: 10.1186/s13287-021-02194-z. Stem Cell Res Ther. 2021. PMID: 33597020 Free PMC article. Review.
-
Restoration of Dystrophin Expression in Mdx-Derived Muscle Progenitor Cells Using CRISPR/Cas9 System and Homology-Directed Repair Technology.Methods Mol Biol. 2023;2587:455-464. doi: 10.1007/978-1-0716-2772-3_23. Methods Mol Biol. 2023. PMID: 36401043
-
Aging-Associated Differences in Epitranscriptomic m6A Regulation in Response to Acute Cardiac Ischemia/Reperfusion Injury in Female Mice.Front Pharmacol. 2021 Aug 3;12:654316. doi: 10.3389/fphar.2021.654316. eCollection 2021. Front Pharmacol. 2021. PMID: 34413770 Free PMC article.
References
Grants and funding
LinkOut - more resources
Full Text Sources