Local Ca2+ signals couple activation of TRPV1 and ANO1 sensory ion channels
- PMID: 32345727
- PMCID: PMC7285899
- DOI: 10.1126/scisignal.aaw7963
Local Ca2+ signals couple activation of TRPV1 and ANO1 sensory ion channels
Abstract
ANO1 (TMEM16A) is a Ca2+-activated Cl- channel (CaCC) expressed in peripheral somatosensory neurons that are activated by painful (noxious) stimuli. These neurons also express the Ca2+-permeable channel and noxious heat sensor TRPV1, which can activate ANO1. Here, we revealed an intricate mechanism of TRPV1-ANO1 channel coupling in rat dorsal root ganglion (DRG) neurons. Simultaneous optical monitoring of CaCC activity and Ca2+ dynamics revealed that the TRPV1 ligand capsaicin activated CaCCs. However, depletion of endoplasmic reticulum (ER) Ca2+ stores reduced capsaicin-induced Ca2+ increases and CaCC activation, suggesting that ER Ca2+ release contributed to TRPV1-induced CaCC activation. ER store depletion by plasma membrane-localized TRPV1 channels was demonstrated with an ER-localized Ca2+ sensor in neurons exposed to a cell-impermeable TRPV1 ligand. Proximity ligation assays established that ANO1, TRPV1, and the IP3 receptor IP3R1 were often found in close proximity to each other. Stochastic optical reconstruction microscopy (STORM) confirmed the close association between all three channels in DRG neurons. Together, our data reveal the existence of ANO1-containing multichannel nanodomains in DRG neurons and suggest that coupling between TRPV1 and ANO1 requires ER Ca2+ release, which may be necessary to enhance ANO1 activation.
Copyright © 2020 The Authors, some rights reserved; exclusive licensee American Association for the Advancement of Science. No claim to original U.S. Government Works.
Conflict of interest statement
Figures
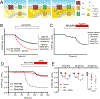
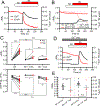
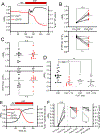
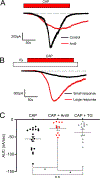
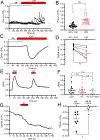
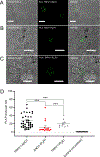
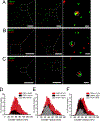
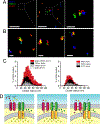
Similar articles
-
The endoplasmic reticulum of dorsal root ganglion neurons contains functional TRPV1 channels.J Biol Chem. 2009 Nov 20;284(47):32591-601. doi: 10.1074/jbc.M109.019687. Epub 2009 Sep 24. J Biol Chem. 2009. PMID: 19778904 Free PMC article.
-
Activation of the Cl- channel ANO1 by localized calcium signals in nociceptive sensory neurons requires coupling with the IP3 receptor.Sci Signal. 2013 Aug 27;6(290):ra73. doi: 10.1126/scisignal.2004184. Sci Signal. 2013. PMID: 23982204 Free PMC article.
-
Anoctamin-1 Cl(-) channels in nociception: activation by an N-aroylaminothiazole and capsaicin and inhibition by T16A[inh]-A01.Mol Pain. 2015 Sep 12;11:55. doi: 10.1186/s12990-015-0061-y. Mol Pain. 2015. PMID: 26364309 Free PMC article.
-
Anoctamin 1, a multi-modal player in pain and itch.Cell Calcium. 2024 Nov;123:102924. doi: 10.1016/j.ceca.2024.102924. Epub 2024 Jun 22. Cell Calcium. 2024. PMID: 38964236 Review.
-
Activation of Ca(2+) -activated Cl(-) channel ANO1 by localized Ca(2+) signals.J Physiol. 2016 Jan 1;594(1):19-30. doi: 10.1113/jphysiol.2014.275107. Epub 2014 Dec 15. J Physiol. 2016. PMID: 25398532 Free PMC article. Review.
Cited by
-
Molecular mechanisms of activation and regulation of ANO1-Encoded Ca2+-Activated Cl- channels.Channels (Austin). 2021 Dec;15(1):569-603. doi: 10.1080/19336950.2021.1975411. Channels (Austin). 2021. PMID: 34488544 Free PMC article. Review.
-
Super-Resolution Analysis of the Origins of the Elementary Events of ER Calcium Release in Dorsal Root Ganglion Neurons.Cells. 2023 Dec 23;13(1):38. doi: 10.3390/cells13010038. Cells. 2023. PMID: 38201242 Free PMC article.
-
Cyst growth in ADPKD is prevented by pharmacological and genetic inhibition of TMEM16A in vivo.Nat Commun. 2020 Aug 28;11(1):4320. doi: 10.1038/s41467-020-18104-5. Nat Commun. 2020. PMID: 32859916 Free PMC article.
-
Reduced Expression of TMEM16A Impairs Nitric Oxide-Dependent Cl- Transport in Retinal Amacrine Cells.Front Cell Neurosci. 2022 Jul 27;16:937060. doi: 10.3389/fncel.2022.937060. eCollection 2022. Front Cell Neurosci. 2022. PMID: 35966201 Free PMC article.
-
Dysregulated Ca2+ signaling, fluid secretion, and mitochondrial function in a mouse model of early Sjögren's disease.Elife. 2024 Sep 11;13:RP97069. doi: 10.7554/eLife.97069. Elife. 2024. PMID: 39259200 Free PMC article.
References
-
- Hartzell C, Putzier I, Arreola J, Calcium-activated chloride channels. Annu Rev Physiol 67, 719–758 (2005). - PubMed
-
- Pedemonte N, Galietta LJ, Structure and function of TMEM16 proteins (anoctamins). Physiol Rev 94, 419–459 (2014). - PubMed
-
- Caputo A, Caci E, Ferrera L, Pedemonte N, Barsanti C, Sondo E, Pfeffer U, Ravazzolo R, Zegarra-Moran O, Galietta LJ, TMEM16A, a membrane protein associated with calcium-dependent chloride channel activity. Science 322, 590–594 (2008). - PubMed
Publication types
MeSH terms
Substances
Grants and funding
LinkOut - more resources
Full Text Sources
Miscellaneous