Glioblastoma in adults: a Society for Neuro-Oncology (SNO) and European Society of Neuro-Oncology (EANO) consensus review on current management and future directions
- PMID: 32328653
- PMCID: PMC7594557
- DOI: 10.1093/neuonc/noaa106
Glioblastoma in adults: a Society for Neuro-Oncology (SNO) and European Society of Neuro-Oncology (EANO) consensus review on current management and future directions
Abstract
Glioblastomas are the most common form of malignant primary brain tumor and an important cause of morbidity and mortality. In recent years there have been important advances in understanding the molecular pathogenesis and biology of these tumors, but this has not translated into significantly improved outcomes for patients. In this consensus review from the Society for Neuro-Oncology (SNO) and the European Association of Neuro-Oncology (EANO), the current management of isocitrate dehydrogenase wildtype (IDHwt) glioblastomas will be discussed. In addition, novel therapies such as targeted molecular therapies, agents targeting DNA damage response and metabolism, immunotherapies, and viral therapies will be reviewed, as well as the current challenges and future directions for research.
Keywords: clinical trials; diagnosis; glioblastoma; therapy.
© The Author(s) 2020. Published by Oxford University Press on behalf of the Society for Neuro-Oncology. All rights reserved. For permissions, please e-mail: journals.permissions@oup.com.
Figures
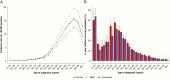
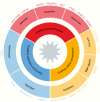
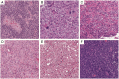
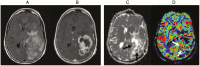
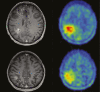
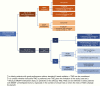
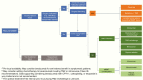
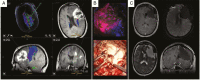
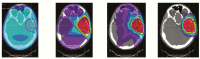
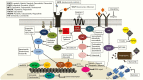
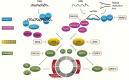
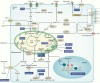
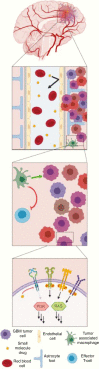
Comment in
-
In the midst of crisis, a great opportunity.Neuro Oncol. 2020 Aug 17;22(8):1056-1057. doi: 10.1093/neuonc/noaa143. Neuro Oncol. 2020. PMID: 32621488 Free PMC article. No abstract available.
-
Role of surgery for glioblastoma: response to letters from Dr. Gerritsen and his colleagues and Dr. Vargas Lopez.Neuro Oncol. 2021 Mar 25;23(3):506-507. doi: 10.1093/neuonc/noaa305. Neuro Oncol. 2021. PMID: 33471080 Free PMC article. No abstract available.
-
Glioblastoma in adults: a Society for Neuro-Oncology (SNO) and European Society of Neuro-Oncology (EANO) consensus review on current management and future directions.Neuro Oncol. 2021 Mar 25;23(3):502-503. doi: 10.1093/neuonc/noaa287. Neuro Oncol. 2021. PMID: 33471898 Free PMC article. No abstract available.
-
Maximizing extent of resection while minimizing the risk of neurological morbidity in glioma patients: a novel grading scale to translate these surgical goals into a merged onco-functional clinical outcome.Neuro Oncol. 2021 Mar 25;23(3):504-505. doi: 10.1093/neuonc/noaa288. Neuro Oncol. 2021. PMID: 33471906 Free PMC article. No abstract available.
Similar articles
-
Isocitrate dehydrogenase (IDH) mutant gliomas: A Society for Neuro-Oncology (SNO) consensus review on diagnosis, management, and future directions.Neuro Oncol. 2023 Jan 5;25(1):4-25. doi: 10.1093/neuonc/noac207. Neuro Oncol. 2023. PMID: 36239925 Free PMC article.
-
Glioblastoma in adults: a Society for Neuro-Oncology (SNO) and European Society of Neuro-Oncology (EANO) consensus review on current management and future directions.Neuro Oncol. 2021 Mar 25;23(3):502-503. doi: 10.1093/neuonc/noaa287. Neuro Oncol. 2021. PMID: 33471898 Free PMC article. No abstract available.
-
Glioblastomas with IDH1/2 mutations have a short clinical history and have a favorable clinical outcome.Jpn J Clin Oncol. 2016 Jan;46(1):31-9. doi: 10.1093/jjco/hyv170. Epub 2015 Nov 24. Jpn J Clin Oncol. 2016. PMID: 26603354
-
Recent developments and future directions in adult lower-grade gliomas: Society for Neuro-Oncology (SNO) and European Association of Neuro-Oncology (EANO) consensus.Neuro Oncol. 2019 Jul 11;21(7):837-853. doi: 10.1093/neuonc/noz033. Neuro Oncol. 2019. PMID: 30753579 Free PMC article. Review.
-
Updates in IDH-Wildtype Glioblastoma.Neurotherapeutics. 2022 Oct;19(6):1705-1723. doi: 10.1007/s13311-022-01251-6. Epub 2022 May 31. Neurotherapeutics. 2022. PMID: 35641844 Free PMC article. Review.
Cited by
-
Tumor Treating Fields for Glioblastoma Therapy During the COVID-19 Pandemic.Front Oncol. 2021 May 7;11:679702. doi: 10.3389/fonc.2021.679702. eCollection 2021. Front Oncol. 2021. PMID: 34026655 Free PMC article.
-
Velcrin molecular glues induce apoptosis in glioblastomas with high PDE3A and SLFN12 expression.Neurooncol Adv. 2024 Jul 1;6(1):vdae115. doi: 10.1093/noajnl/vdae115. eCollection 2024 Jan-Dec. Neurooncol Adv. 2024. PMID: 39166256 Free PMC article.
-
A novel compound EPIC-0412 reverses temozolomide resistance via inhibiting DNA repair/MGMT in glioblastoma.Neuro Oncol. 2023 May 4;25(5):857-870. doi: 10.1093/neuonc/noac242. Neuro Oncol. 2023. PMID: 36272139 Free PMC article.
-
Imaging in patients with glioblastoma: A national cohort study.Neurooncol Pract. 2022 Jun 11;9(6):487-495. doi: 10.1093/nop/npac048. eCollection 2022 Dec. Neurooncol Pract. 2022. PMID: 36381650 Free PMC article.
-
Nuclear Glycoprotein A Repetitions Predominant (GARP) Is a Common Trait of Glioblastoma Stem-like Cells and Correlates with Poor Survival in Glioblastoma Patients.Cancers (Basel). 2023 Dec 5;15(24):5711. doi: 10.3390/cancers15245711. Cancers (Basel). 2023. PMID: 38136258 Free PMC article.
References
-
- Louis DN, Perry A, Reifenberger G, et al. . The 2016 World Health Organization Classification of Tumors of the Central Nervous System: a summary. Acta Neuropathol. 2016;131(6):803–820. - PubMed
Publication types
MeSH terms
Substances
Grants and funding
LinkOut - more resources
Full Text Sources
Other Literature Sources
Medical