ATO (Arsenic Trioxide) Effects on Promyelocytic Leukemia Nuclear Bodies Reveals Antiviral Intervention Capacity
- PMID: 32328411
- PMCID: PMC7175289
- DOI: 10.1002/advs.201902130
ATO (Arsenic Trioxide) Effects on Promyelocytic Leukemia Nuclear Bodies Reveals Antiviral Intervention Capacity
Abstract
Human adenoviruses (HAdV) are associated with clinical symptoms such as gastroenteritis, keratoconjunctivitis, pneumonia, hepatitis, and encephalitis. In the absence of protective immunity, as in allogeneic bone marrow transplant patients, HAdV infections can become lethal. Alarmingly, various outbreaks of highly pathogenic, pneumotropic HAdV types have been recently reported, causing severe and lethal respiratory diseases. Effective drugs for treatment of HAdV infections are still lacking. The repurposing of drugs approved for other indications is a valuable alternative for the development of new antiviral therapies and is less risky and costly than de novo development. Arsenic trioxide (ATO) is approved for treatment of acute promyelocytic leukemia. Here, it is shown that ATO is a potent inhibitor of HAdV. ATO treatment blocks virus expression and replication by reducing the number and integrity of promyelocytic leukemia (PML) nuclear bodies, important subnuclear structures for HAdV replication. Modification of HAdV proteins with small ubiquitin-like modifiers (SUMO) is also key to HAdV replication. ATO reduces levels of viral SUMO-E2A protein, while increasing SUMO-PML, suggesting that ATO interferes with SUMOylation of proteins crucial for HAdV replication. It is concluded that ATO targets cellular processes key to HAdV replication and is relevant for the development of antiviral intervention strategies.
Keywords: antivirals; arsenic; human adenoviruses; promyelocytic leukemia nuclear bodies; small ubiquitin‐like modifiers (SUMO).
© 2020 The Authors. Published by WILEY‐VCH Verlag GmbH & Co. KGaA, Weinheim.
Conflict of interest statement
The authors declare no conflict of interest.
Figures
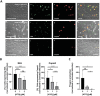
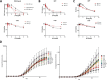
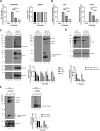
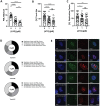
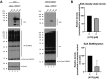
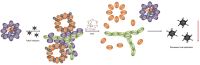
Similar articles
-
Arsenic trioxide impacts hepatitis B virus core nuclear localization and efficiently interferes with HBV infection.Microbiol Spectr. 2024 May 2;12(5):e0378823. doi: 10.1128/spectrum.03788-23. Epub 2024 Apr 3. Microbiol Spectr. 2024. PMID: 38567974 Free PMC article.
-
Viral DNA Binding Protein SUMOylation Promotes PML Nuclear Body Localization Next to Viral Replication Centers.mBio. 2020 Mar 17;11(2):e00049-20. doi: 10.1128/mBio.00049-20. mBio. 2020. PMID: 32184235 Free PMC article.
-
E1B-55K-Mediated Regulation of RNF4 SUMO-Targeted Ubiquitin Ligase Promotes Human Adenovirus Gene Expression.J Virol. 2018 Jun 13;92(13):e00164-18. doi: 10.1128/JVI.00164-18. Print 2018 Jul 1. J Virol. 2018. PMID: 29695423 Free PMC article.
-
A Tale of Usurpation and Subversion: SUMO-Dependent Integrity of Promyelocytic Leukemia Nuclear Bodies at the Crossroad of Infection and Immunity.Front Cell Dev Biol. 2021 Aug 27;9:696234. doi: 10.3389/fcell.2021.696234. eCollection 2021. Front Cell Dev Biol. 2021. PMID: 34513832 Free PMC article. Review.
-
Double-edged role of PML nuclear bodies during human adenovirus infection.Virus Res. 2021 Apr 2;295:198280. doi: 10.1016/j.virusres.2020.198280. Epub 2020 Dec 25. Virus Res. 2021. PMID: 33370557 Review.
Cited by
-
PML Alternative Splice Products Differentially Regulate HAdV Productive Infection.Microbiol Spectr. 2022 Aug 31;10(4):e0078522. doi: 10.1128/spectrum.00785-22. Epub 2022 Jun 14. Microbiol Spectr. 2022. PMID: 35699431 Free PMC article.
-
Arsenic trioxide impacts hepatitis B virus core nuclear localization and efficiently interferes with HBV infection.Microbiol Spectr. 2024 May 2;12(5):e0378823. doi: 10.1128/spectrum.03788-23. Epub 2024 Apr 3. Microbiol Spectr. 2024. PMID: 38567974 Free PMC article.
-
Arsenic trioxide: applications, mechanisms of action, toxicity and rescue strategies to date.Arch Pharm Res. 2024 Mar;47(3):249-271. doi: 10.1007/s12272-023-01481-y. Epub 2023 Dec 26. Arch Pharm Res. 2024. PMID: 38147202 Review.
-
Arsenic in medicine: past, present and future.Biometals. 2023 Apr;36(2):283-301. doi: 10.1007/s10534-022-00371-y. Epub 2022 Feb 21. Biometals. 2023. PMID: 35190937 Free PMC article. Review.
-
Multiple roles of arsenic compounds in phase separation and membraneless organelles formation determine their therapeutic efficacy in tumors.J Pharm Anal. 2024 Aug;14(8):100957. doi: 10.1016/j.jpha.2024.02.011. Epub 2024 Feb 24. J Pharm Anal. 2024. PMID: 39253293 Free PMC article. Review.
References
LinkOut - more resources
Full Text Sources
Other Literature Sources