Oligomerization of the Vesicular Stomatitis Virus Phosphoprotein Is Dispensable for mRNA Synthesis but Facilitates RNA Replication
- PMID: 32321813
- PMCID: PMC7307139
- DOI: 10.1128/JVI.00115-20
Oligomerization of the Vesicular Stomatitis Virus Phosphoprotein Is Dispensable for mRNA Synthesis but Facilitates RNA Replication
Abstract
Nonsegmented negative-strand (NNS) RNA viruses possess a ribonucleoprotein template in which the genomic RNA is sequestered within a homopolymer of nucleocapsid protein (N). The viral RNA-dependent RNA polymerase (RdRP) resides within an approximately 250-kDa large protein (L), along with unconventional mRNA capping enzymes: a GDP:polyribonucleotidyltransferase (PRNT) and a dual-specificity mRNA cap methylase (MT). To gain access to the N-RNA template and orchestrate the LRdRP, LPRNT, and LMT, an oligomeric phosphoprotein (P) is required. Vesicular stomatitis virus (VSV) P is dimeric with an oligomerization domain (OD) separating two largely disordered regions followed by a globular C-terminal domain that binds the template. P is also responsible for bringing new N protomers onto the nascent RNA during genome replication. We show VSV P lacking the OD (PΔOD) is monomeric but is indistinguishable from wild-type P in supporting mRNA transcription in vitro Recombinant virus VSV-PΔOD exhibits a pronounced kinetic delay in progeny virus production. Fluorescence recovery after photobleaching demonstrates that PΔOD diffuses 6-fold more rapidly than the wild type within viral replication compartments. A well-characterized defective interfering particle of VSV (DI-T) that is only competent for RNA replication requires significantly higher levels of N to drive RNA replication in the presence of PΔOD We conclude P oligomerization is not required for mRNA synthesis but enhances genome replication by facilitating RNA encapsidation.IMPORTANCE All NNS RNA viruses, including the human pathogens rabies, measles, respiratory syncytial virus, Nipah, and Ebola, possess an essential L-protein cofactor, required to access the N-RNA template and coordinate the various enzymatic activities of L. The polymerase cofactors share a similar modular organization of a soluble N-binding domain and a template-binding domain separated by a central oligomerization domain. Using a prototype of NNS RNA virus gene expression, vesicular stomatitis virus (VSV), we determined the importance of P oligomerization. We find that oligomerization of VSV P is not required for any step of viral mRNA synthesis but is required for efficient RNA replication. We present evidence that this likely occurs through the stage of loading soluble N onto the nascent RNA strand as it exits the polymerase during RNA replication. Interfering with the oligomerization of P may represent a general strategy to interfere with NNS RNA virus replication.
Keywords: genome replication; oligomerization; phosphoprotein; polymerase; vesicular stomatitis virus.
Copyright © 2020 Bloyet et al.
Figures
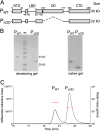
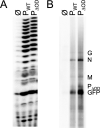
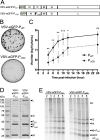
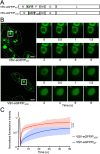
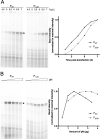
Similar articles
-
An In Vitro RNA Synthesis Assay for Rabies Virus Defines Ribonucleoprotein Interactions Critical for Polymerase Activity.J Virol. 2016 Dec 16;91(1):e01508-16. doi: 10.1128/JVI.01508-16. Print 2017 Jan 1. J Virol. 2016. PMID: 27795419 Free PMC article.
-
Vesicular Stomatitis Virus Phosphoprotein Dimerization Domain Is Dispensable for Virus Growth.J Virol. 2020 Feb 28;94(6):e01789-19. doi: 10.1128/JVI.01789-19. Print 2020 Feb 28. J Virol. 2020. PMID: 31852780 Free PMC article.
-
A freeze frame view of vesicular stomatitis virus transcription defines a minimal length of RNA for 5' processing.PLoS Pathog. 2011 Jun;7(6):e1002073. doi: 10.1371/journal.ppat.1002073. Epub 2011 Jun 2. PLoS Pathog. 2011. PMID: 21655110 Free PMC article.
-
An unconventional pathway of mRNA cap formation by vesiculoviruses.Virus Res. 2011 Dec;162(1-2):100-9. doi: 10.1016/j.virusres.2011.09.012. Epub 2011 Sep 16. Virus Res. 2011. PMID: 21945214 Free PMC article. Review.
-
Structures of the Mononegavirales Polymerases.J Virol. 2020 Oct 27;94(22):e00175-20. doi: 10.1128/JVI.00175-20. Print 2020 Oct 27. J Virol. 2020. PMID: 32847861 Free PMC article. Review.
Cited by
-
The Nucleocapsid of Paramyxoviruses: Structure and Function of an Encapsidated Template.Viruses. 2021 Dec 9;13(12):2465. doi: 10.3390/v13122465. Viruses. 2021. PMID: 34960734 Free PMC article. Review.
-
Predicting substitutions to modulate disorder and stability in coiled-coils.BMC Bioinformatics. 2020 Dec 21;21(Suppl 19):573. doi: 10.1186/s12859-020-03867-x. BMC Bioinformatics. 2020. PMID: 33349244 Free PMC article.
-
Stochastic model of vesicular stomatitis virus replication reveals mutational effects on virion production.PLoS Comput Biol. 2024 Feb 7;20(2):e1011373. doi: 10.1371/journal.pcbi.1011373. eCollection 2024 Feb. PLoS Comput Biol. 2024. PMID: 38324583 Free PMC article.
-
Specific Residues in the C-Terminal Domain of the Human Metapneumovirus Phosphoprotein Are Indispensable for Formation of Viral Replication Centers and Regulation of the Function of the Viral Polymerase Complex.J Virol. 2023 May 31;97(5):e0003023. doi: 10.1128/jvi.00030-23. Epub 2023 Apr 24. J Virol. 2023. PMID: 37092993 Free PMC article.
References
Publication types
MeSH terms
Substances
Grants and funding
LinkOut - more resources
Full Text Sources
Research Materials
Miscellaneous