The Set7 Lysine Methyltransferase Regulates Plasticity in Oxidative Phosphorylation Necessary for Trained Immunity Induced by β-Glucan
- PMID: 32320649
- PMCID: PMC7184679
- DOI: 10.1016/j.celrep.2020.107548
The Set7 Lysine Methyltransferase Regulates Plasticity in Oxidative Phosphorylation Necessary for Trained Immunity Induced by β-Glucan
Abstract
Trained immunity confers a sustained augmented response of innate immune cells to a secondary challenge, via a process dependent on metabolic and transcriptional reprogramming. Because of its previous associations with metabolic and transcriptional memory, as well as the importance of H3 histone lysine 4 monomethylation (H3K4me1) to innate immune memory, we hypothesize that the Set7 methyltransferase has an important role in trained immunity induced by β-glucan. Using pharmacological studies of human primary monocytes, we identify trained immunity-specific immunometabolic pathways regulated by Set7, including a previously unreported H3K4me1-dependent plasticity in the induction of oxidative phosphorylation. Recapitulation of β-glucan training in vivo additionally identifies Set7-dependent changes in gene expression previously associated with the modulation of myelopoiesis progenitors in trained immunity. By revealing Set7 as a key regulator of trained immunity, these findings provide mechanistic insight into sustained metabolic changes and underscore the importance of characterizing regulatory circuits of innate immune memory.
Keywords: Set7; immunometabolism; inflammation; macrophage; methylation; monocyte; oxidative phosphorylation; trained immunity; β-glucan.
Copyright © 2020 The Author(s). Published by Elsevier Inc. All rights reserved.
Conflict of interest statement
Declaration of Interests W.J.H.K. is a scientific advisor of Khondrion (Nijmegen, the Netherlands) and of Fortify Therapeutics. These subject matter experts had no involvement in the data collection, analysis and interpretation, writing of the manuscript, and the decision to submit the manuscript for publication.
Figures
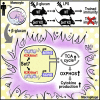
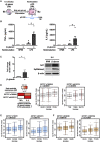
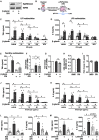
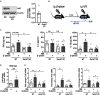
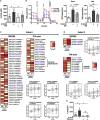
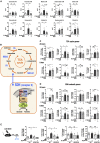
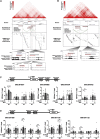
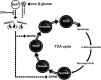
Similar articles
-
Transcriptional regulation by the Set7 lysine methyltransferase.Epigenetics. 2013 Apr;8(4):361-72. doi: 10.4161/epi.24234. Epub 2013 Mar 11. Epigenetics. 2013. PMID: 23478572 Free PMC article. Review.
-
Transcriptional activity of the islet β cell factor Pdx1 is augmented by lysine methylation catalyzed by the methyltransferase Set7/9.J Biol Chem. 2015 Apr 10;290(15):9812-22. doi: 10.1074/jbc.M114.616219. Epub 2015 Feb 24. J Biol Chem. 2015. PMID: 25713082 Free PMC article.
-
Immunometabolic changes of β-glucan-trained immunity induction and inhibition on neonatal calf immune innate cells.Mol Immunol. 2023 Jul;159:58-68. doi: 10.1016/j.molimm.2023.05.008. Epub 2023 Jun 2. Mol Immunol. 2023. PMID: 37271010
-
Screening of compounds to identify novel epigenetic regulatory factors that affect innate immune memory in macrophages.Sci Rep. 2022 Feb 3;12(1):1912. doi: 10.1038/s41598-022-05929-x. Sci Rep. 2022. PMID: 35115604 Free PMC article.
-
SET7, a lysine-specific methyl transferase: An intriguing epigenetic target to combat diabetic nephropathy.Drug Discov Today. 2023 Oct;28(10):103754. doi: 10.1016/j.drudis.2023.103754. Epub 2023 Aug 28. Drug Discov Today. 2023. PMID: 37648018 Review.
Cited by
-
Impact of Immunity on Coronary Artery Disease: An Updated Pathogenic Interplay and Potential Therapeutic Strategies.Life (Basel). 2023 Oct 27;13(11):2128. doi: 10.3390/life13112128. Life (Basel). 2023. PMID: 38004268 Free PMC article. Review.
-
β-glucans from Agaricus bisporus mushroom products drive Trained Immunity.Front Nutr. 2024 Feb 15;11:1346706. doi: 10.3389/fnut.2024.1346706. eCollection 2024. Front Nutr. 2024. PMID: 38425482 Free PMC article.
-
Trained Immunity and Reactivity of Macrophages and Endothelial Cells.Arterioscler Thromb Vasc Biol. 2021 Mar;41(3):1032-1046. doi: 10.1161/ATVBAHA.120.315452. Epub 2020 Dec 31. Arterioscler Thromb Vasc Biol. 2021. PMID: 33380171 Free PMC article. Review.
-
Lysine methyltransferase G9a is an important modulator of trained immunity.Clin Transl Immunology. 2021 Feb 18;10(2):e1253. doi: 10.1002/cti2.1253. eCollection 2021. Clin Transl Immunology. 2021. PMID: 33708384 Free PMC article.
-
The mechanisms and cross-protection of trained innate immunity.Virol J. 2022 Dec 8;19(1):210. doi: 10.1186/s12985-022-01937-5. Virol J. 2022. PMID: 36482472 Free PMC article. Review.
References
-
- Adams D., Altucci L., Antonarakis S.E., Ballesteros J., Beck S., Bird A., Bock C., Boehm B., Campo E., Caricasole A. BLUEPRINT to decode the epigenetic signature written in blood. Nat. Biotechnol. 2012;30:224–226. - PubMed
-
- Allis C.D., Berger S.L., Cote J., Dent S., Jenuwien T., Kouzarides T., Pillus L., Reinberg D., Shi Y., Shiekhattar R. New nomenclature for chromatin-modifying enzymes. Cell. 2007;131:633–636. - PubMed
-
- Bekkering S., Quintin J., Joosten L.A., van der Meer J.W., Netea M.G., Riksen N.P. Oxidized low-density lipoprotein induces long-term proinflammatory cytokine production and foam cell formation via epigenetic reprogramming of monocytes. Arterioscler. Thromb. Vasc. Biol. 2014;34:1731–1738. - PubMed
Publication types
MeSH terms
Substances
LinkOut - more resources
Full Text Sources
Molecular Biology Databases