Applications of genome editing technology in the targeted therapy of human diseases: mechanisms, advances and prospects
- PMID: 32296011
- PMCID: PMC6946647
- DOI: 10.1038/s41392-019-0089-y
Applications of genome editing technology in the targeted therapy of human diseases: mechanisms, advances and prospects
Abstract
Based on engineered or bacterial nucleases, the development of genome editing technologies has opened up the possibility of directly targeting and modifying genomic sequences in almost all eukaryotic cells. Genome editing has extended our ability to elucidate the contribution of genetics to disease by promoting the creation of more accurate cellular and animal models of pathological processes and has begun to show extraordinary potential in a variety of fields, ranging from basic research to applied biotechnology and biomedical research. Recent progress in developing programmable nucleases, such as zinc-finger nucleases (ZFNs), transcription activator-like effector nucleases (TALENs) and clustered regularly interspaced short palindromic repeat (CRISPR)-Cas-associated nucleases, has greatly expedited the progress of gene editing from concept to clinical practice. Here, we review recent advances of the three major genome editing technologies (ZFNs, TALENs, and CRISPR/Cas9) and discuss the applications of their derivative reagents as gene editing tools in various human diseases and potential future therapies, focusing on eukaryotic cells and animal models. Finally, we provide an overview of the clinical trials applying genome editing platforms for disease treatment and some of the challenges in the implementation of this technology.
Conflict of interest statement
The authors declare no competing interests.
Figures
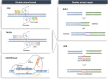
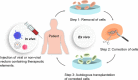
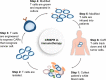
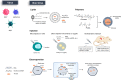
Similar articles
-
Therapeutic Genome Editing and In Vivo Delivery.AAPS J. 2021 Jun 2;23(4):80. doi: 10.1208/s12248-021-00613-w. AAPS J. 2021. PMID: 34080099 Review.
-
Recent Advances in Therapeutic Genome Editing in China.Hum Gene Ther. 2018 Feb;29(2):136-145. doi: 10.1089/hum.2017.210. Hum Gene Ther. 2018. PMID: 29446996 Review.
-
CRISPR/Cas9: an advanced tool for editing plant genomes.Transgenic Res. 2016 Oct;25(5):561-73. doi: 10.1007/s11248-016-9953-5. Epub 2016 Mar 24. Transgenic Res. 2016. PMID: 27012546 Review.
-
Progress in and Prospects of Genome Editing Tools for Human Disease Model Development and Therapeutic Applications.Genes (Basel). 2023 Feb 14;14(2):483. doi: 10.3390/genes14020483. Genes (Basel). 2023. PMID: 36833410 Free PMC article. Review.
-
A Review of CRISPR-Based Genome Editing: Survival, Evolution and Challenges.Curr Issues Mol Biol. 2018;28:47-68. doi: 10.21775/cimb.028.047. Epub 2018 Feb 11. Curr Issues Mol Biol. 2018. PMID: 29428910 Review.
Cited by
-
Nanogel: A versatile drug delivery system for the treatment of various diseases and their future perspective.Drug Deliv Transl Res. 2025 Feb;15(2):455-482. doi: 10.1007/s13346-024-01684-w. Epub 2024 Aug 5. Drug Deliv Transl Res. 2025. PMID: 39103593 Review.
-
Codon usage and expression-based features significantly improve prediction of CRISPR efficiency.NPJ Syst Biol Appl. 2024 Sep 3;10(1):100. doi: 10.1038/s41540-024-00431-8. NPJ Syst Biol Appl. 2024. PMID: 39227603 Free PMC article.
-
Advances in Type 1 Diabetes Mellitus Management in Children.Cureus. 2024 Aug 21;16(8):e67377. doi: 10.7759/cureus.67377. eCollection 2024 Aug. Cureus. 2024. PMID: 39310514 Free PMC article. Review.
-
Genome-editing approaches and applications: a brief review on CRISPR technology and its role in cancer.3 Biotech. 2021 Mar;11(3):146. doi: 10.1007/s13205-021-02680-4. Epub 2021 Feb 26. 3 Biotech. 2021. PMID: 33732568 Free PMC article. Review.
-
Precision in Action: The Role of Clustered Regularly Interspaced Short Palindromic Repeats/Cas in Gene Therapies.Vaccines (Basel). 2024 Jun 7;12(6):636. doi: 10.3390/vaccines12060636. Vaccines (Basel). 2024. PMID: 38932365 Free PMC article. Review.
References
Publication types
MeSH terms
Grants and funding
LinkOut - more resources
Full Text Sources
Other Literature Sources
Medical