Disruption of genes associated with Charcot-Marie-Tooth type 2 lead to common behavioural, cellular and molecular defects in Caenorhabditis elegans
- PMID: 32294113
- PMCID: PMC7159224
- DOI: 10.1371/journal.pone.0231600
Disruption of genes associated with Charcot-Marie-Tooth type 2 lead to common behavioural, cellular and molecular defects in Caenorhabditis elegans
Abstract
Charcot-Marie-Tooth (CMT) disease is an inherited peripheral motor and sensory neuropathy. The disease is divided into demyelinating (CMT1) and axonal (CMT2) neuropathies, and although we have gained molecular information into the details of CMT1 pathology, much less is known about CMT2. Due to its clinical and genetic heterogeneity, coupled with a lack of animal models, common underlying mechanisms remain elusive. In order to gain an understanding of the normal function of genes associated with CMT2, and to draw direct comparisons between them, we have studied the behavioural, cellular and molecular consequences of mutating nine different genes in the nematode Caenorhabditis elegans (lin-41/TRIM2, dyn-1/DNM2, unc-116/KIF5A, fzo-1/MFN2, osm-9/TRPV4, cua-1/ATP7A, hsp-25/HSPB1, hint-1/HINT1, nep-2/MME). We show that C. elegans defective for these genes display debilitated movement in crawling and swimming assays. Severe morphological defects in cholinergic motors neurons are also evident in two of the mutants (dyn-1 and unc-116). Furthermore, we establish methods for quantifying muscle morphology and use these to demonstrate that loss of muscle structure occurs in the majority of mutants studied. Finally, using electrophysiological recordings of neuromuscular junction (NMJ) activity, we uncover reductions in spontaneous postsynaptic current frequency in lin-41, dyn-1, unc-116 and fzo-1 mutants. By comparing the consequences of mutating numerous CMT2-related genes, this study reveals common deficits in muscle structure and function, as well as NMJ signalling when these genes are disrupted.
Conflict of interest statement
The authors have declared that no competing interests exist.
Figures
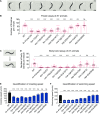
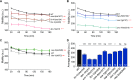
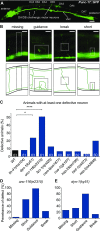
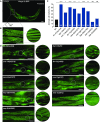
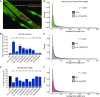
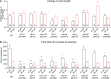
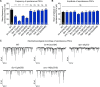
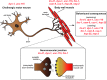
Similar articles
-
Genetic epidemiology of Charcot-Marie-Tooth disease.Acta Neurol Scand Suppl. 2012;(193):iv-22. doi: 10.1111/ane.12013. Acta Neurol Scand Suppl. 2012. PMID: 23106488
-
Synaptic Deficits at Neuromuscular Junctions in Two Mouse Models of Charcot-Marie-Tooth Type 2d.J Neurosci. 2016 Mar 16;36(11):3254-67. doi: 10.1523/JNEUROSCI.1762-15.2016. J Neurosci. 2016. PMID: 26985035 Free PMC article.
-
Axonal Transport Defects in a Mitofusin 2 Loss of Function Model of Charcot-Marie-Tooth Disease in Zebrafish.PLoS One. 2013 Jun 26;8(6):e67276. doi: 10.1371/journal.pone.0067276. Print 2013. PLoS One. 2013. PMID: 23840650 Free PMC article.
-
[Molecular genetics of inherited neuropathies].Rinsho Shinkeigaku. 2006 Jan;46(1):1-18. Rinsho Shinkeigaku. 2006. PMID: 16541790 Review. Japanese.
-
Intermediate Charcot-Marie-Tooth disease: an electrophysiological reappraisal and systematic review.J Neurol. 2017 Aug;264(8):1655-1677. doi: 10.1007/s00415-017-8474-3. Epub 2017 Mar 31. J Neurol. 2017. PMID: 28364294 Review.
Cited by
-
TRPV4: A Physio and Pathophysiologically Significant Ion Channel.Int J Mol Sci. 2020 May 28;21(11):3837. doi: 10.3390/ijms21113837. Int J Mol Sci. 2020. PMID: 32481620 Free PMC article. Review.
-
Charcot-Marie-tooth disease causing mutation (p.R158H) in pyruvate dehydrogenase kinase 3 (PDK3) affects synaptic transmission, ATP production and causes neurodegeneration in a CMTX6 C. elegans model.Hum Mol Genet. 2021 Dec 17;31(1):133-145. doi: 10.1093/hmg/ddab228. Hum Mol Genet. 2021. PMID: 34387338 Free PMC article.
-
Developmental demands contribute to early neuromuscular degeneration in CMT2D mice.Cell Death Dis. 2020 Jul 23;11(7):564. doi: 10.1038/s41419-020-02798-y. Cell Death Dis. 2020. PMID: 32703932 Free PMC article.
-
The M1311V variant of ATP7A is associated with impaired trafficking and copper homeostasis in models of motor neuron disease.Neurobiol Dis. 2021 Feb;149:105228. doi: 10.1016/j.nbd.2020.105228. Epub 2020 Dec 24. Neurobiol Dis. 2021. PMID: 33359139 Free PMC article.
-
Exploring the putative microRNAs cross-kingdom transfer in Solanum lycopersicum-Meloidogyne incognita interactions.Front Plant Sci. 2024 May 8;15:1383986. doi: 10.3389/fpls.2024.1383986. eCollection 2024. Front Plant Sci. 2024. PMID: 38784062 Free PMC article.
References
-
- Barisic N, Claeys KG, Sirotković-Skerlev M, Löfgren A, Nelis E, De Jonghe P, et al. Charcot-Marie-Tooth disease: A clinico-genetic confrontation. Ann Hum Genet. 2007;72(3):416–41. - PubMed
Publication types
MeSH terms
Substances
Grants and funding
LinkOut - more resources
Full Text Sources
Other Literature Sources
Medical
Research Materials
Miscellaneous