Electrical stimulation of cranial nerves in cognition and disease
- PMID: 32289703
- PMCID: PMC7196013
- DOI: 10.1016/j.brs.2020.02.019
Electrical stimulation of cranial nerves in cognition and disease
Abstract
The cranial nerves are the pathways through which environmental information (sensation) is directly communicated to the brain, leading to perception, and giving rise to higher cognition. Because cranial nerves determine and modulate brain function, invasive and non-invasive cranial nerve electrical stimulation methods have applications in the clinical, behavioral, and cognitive domains. Among other neuromodulation approaches such as peripheral, transcranial and deep brain stimulation, cranial nerve stimulation is unique in allowing axon pathway-specific engagement of brain circuits, including thalamo-cortical networks. In this review we amalgamate relevant knowledge of 1) cranial nerve anatomy and biophysics; 2) evidence of the modulatory effects of cranial nerves on cognition; 3) clinical and behavioral outcomes of cranial nerve stimulation; and 4) biomarkers of nerve target engagement including physiology, electroencephalography, neuroimaging, and behavioral metrics. Existing non-invasive stimulation methods cannot feasibly activate the axons of only individual cranial nerves. Even with invasive stimulation methods, selective targeting of one nerve fiber type requires nuance since each nerve is composed of functionally distinct axon-types that differentially branch and can anastomose onto other nerves. None-the-less, precisely controlling stimulation parameters can aid in affecting distinct sets of axons, thus supporting specific actions on cognition and behavior. To this end, a rubric for reproducible dose-response stimulation parameters is defined here. Given that afferent cranial nerve axons project directly to the brain, targeting structures (e.g. thalamus, cortex) that are critical nodes in higher order brain networks, potent effects on cognition are plausible. We propose an intervention design framework based on driving cranial nerve pathways in targeted brain circuits, which are in turn linked to specific higher cognitive processes. State-of-the-art current flow models that are used to explain and design cranial-nerve-activating stimulation technology require multi-scale detail that includes: gross anatomy; skull foramina and superficial tissue layers; and precise nerve morphology. Detailed simulations also predict that some non-invasive electrical or magnetic stimulation approaches that do not intend to modulate cranial nerves per se, such as transcranial direct current stimulation (tDCS) and transcranial magnetic stimulation (TMS), may also modulate activity of specific cranial nerves. Much prior cranial nerve stimulation work was conceptually limited to the production of sensory perception, with individual titration of intensity based on the level of perception and tolerability. However, disregarding sensory emulation allows consideration of temporal stimulation patterns (axon recruitment) that modulate the tone of cortical networks independent of sensory cortices, without necessarily titrating perception. For example, leveraging the role of the thalamus as a gatekeeper for information to the cerebral cortex, preventing or enhancing the passage of specific information depending on the behavioral state. We show that properly parameterized computational models at multiple scales are needed to rationally optimize neuromodulation that target sets of cranial nerves, determining which and how specific brain circuitries are modulated, which can in turn influence cognition in a designed manner.
Keywords: Cranial nerve; Olfactory; Optic; Stimulation; Trigeminal; Vagus; Vestibulocochlear.
Copyright © 2020 The Author(s). Published by Elsevier Inc. All rights reserved.
Conflict of interest statement
Declaration of competing interest The City University of New York has inventions on tES with MB as inventor. MB has equity in Soterix Medical and serves on the scientific advisory boards or has grants from Mecta, Halo Neuroscience, Boston Scientific and GlaxoSmithKline. BWB is an inventor on tES patents and patent applications, has equity in Bodhi NeuroTech and serves as a consultant to eQuility. Vitaly Napadow has a financial interest in Cala Health which is licensing taVNS technology from Massachusetts General Hospital, and his interest was reviewed and managed by the Massachusetts General Hospital and Partners HealthCare in accordance with their institutional policies. JDB has research grant support from ElectroCore, Inc. The rest of co-authors have nothing to disclose.
Figures
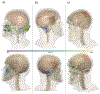
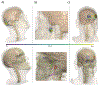
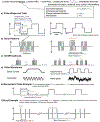
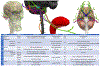
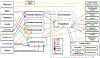
Similar articles
-
Neuroimaging and neuromodulation approaches to study eating behavior and prevent and treat eating disorders and obesity.Neuroimage Clin. 2015 Mar 24;8:1-31. doi: 10.1016/j.nicl.2015.03.016. eCollection 2015. Neuroimage Clin. 2015. PMID: 26110109 Free PMC article. Review.
-
Do tDCS and TMS influence tinnitus transiently via a direct cortical and indirect somatosensory modulating effect? A combined TMS-tDCS and TENS study.Brain Stimul. 2011 Oct;4(4):242-52. doi: 10.1016/j.brs.2010.12.001. Epub 2011 Jan 1. Brain Stimul. 2011. PMID: 22032739 Clinical Trial.
-
Incomplete evidence that increasing current intensity of tDCS boosts outcomes.Brain Stimul. 2018 Mar-Apr;11(2):310-321. doi: 10.1016/j.brs.2017.12.002. Epub 2017 Dec 13. Brain Stimul. 2018. PMID: 29258808 Free PMC article. Review.
-
Vision modulation, plasticity and restoration using non-invasive brain stimulation - An IFCN-sponsored review.Clin Neurophysiol. 2020 Apr;131(4):887-911. doi: 10.1016/j.clinph.2020.01.008. Epub 2020 Feb 3. Clin Neurophysiol. 2020. PMID: 32078919 Review.
-
Non-invasive brain stimulation techniques for chronic pain.Cochrane Database Syst Rev. 2018 Apr 13;4(4):CD008208. doi: 10.1002/14651858.CD008208.pub5. Cochrane Database Syst Rev. 2018. PMID: 29652088 Free PMC article. Review.
Cited by
-
Transcutaneous auricular vagus nerve stimulation induces stabilizing modifications in large-scale functional brain networks: towards understanding the effects of taVNS in subjects with epilepsy.Sci Rep. 2021 Apr 12;11(1):7906. doi: 10.1038/s41598-021-87032-1. Sci Rep. 2021. PMID: 33846432 Free PMC article. Clinical Trial.
-
No Impact of Stochastic Galvanic Vestibular Stimulation on Arterial Pressure and Heart Rate Variability in the Elderly Population.Front Hum Neurosci. 2021 Feb 17;15:646127. doi: 10.3389/fnhum.2021.646127. eCollection 2021. Front Hum Neurosci. 2021. PMID: 33679355 Free PMC article.
-
Review of Noninvasive or Minimally Invasive Deep Brain Stimulation.Front Behav Neurosci. 2022 Jan 18;15:820017. doi: 10.3389/fnbeh.2021.820017. eCollection 2021. Front Behav Neurosci. 2022. PMID: 35145384 Free PMC article. Review.
-
Assessing the Effect of Simultaneous Combining of Transcranial Direct Current Stimulation and Transcutaneous Auricular Vagus Nerve Stimulation on the Improvement of Working Memory Performance in Healthy Individuals.Front Neurosci. 2022 Jul 19;16:947236. doi: 10.3389/fnins.2022.947236. eCollection 2022. Front Neurosci. 2022. PMID: 35928012 Free PMC article.
-
Effect of non-invasive neuromodulation techniques on vascular cognitive impairment: A Bayesian network meta-analysis protocol.PLoS One. 2024 Jan 4;19(1):e0284447. doi: 10.1371/journal.pone.0284447. eCollection 2024. PLoS One. 2024. PMID: 38175852 Free PMC article.
References
-
- Kandel ER, Schwartz JH, Jessell TM, Siegelbaum SA, Hudspeth AJ. Principles of neural science: McGraw-hill; New York; 2000.
-
- Parvizi J, Van Hoesen GW, Damasio A. The selective vulnerability of brainstem nuclei to Alzheimer’s disease. Annals of neurology 2001;49(1):53–66. - PubMed
-
- DeGiorgio CM, Fanselow EE, Schrader LM, Cook IA. Trigeminal nerve stimulation: seminal animal and human studies for epilepsy and depression. Neurosurgery clinics of North America 2011;22(4):449–56. - PubMed
-
- Hanes DA, McCollum G. Cognitive-vestibular interactions: a review of patient difficulties and possible mechanisms. Journal of Vestibular Research 2006;16(3):75–91. - PubMed
-
- Rea P Clinical anatomy of the cranial nerves: Academic Press; 2014.
Publication types
MeSH terms
Grants and funding
- I01 RX003418/RX/RRD VA/United States
- P30 GM122734/GM/NIGMS NIH HHS/United States
- P01 AT009965/AT/NCCIH NIH HHS/United States
- R01 MH120262/MH/NIMH NIH HHS/United States
- P2C HD086844/HD/NICHD NIH HHS/United States
- OT2 OD023867/OD/NIH HHS/United States
- R01 NS101362/NS/NINDS NIH HHS/United States
- UG3 DA048502/DA/NIDA NIH HHS/United States
- R21 MH103468/MH/NIMH NIH HHS/United States
- U54 CA137788/CA/NCI NIH HHS/United States
- S10 RR016917/RR/NCRR NIH HHS/United States
- T32 MH067547/MH/NIMH NIH HHS/United States
- R01 MH111896/MH/NIMH NIH HHS/United States
- U54 CA132378/CA/NCI NIH HHS/United States
- T32 GM136499/GM/NIGMS NIH HHS/United States
- K24 MH076955/MH/NIMH NIH HHS/United States
- R03 NS054783/NS/NINDS NIH HHS/United States
LinkOut - more resources
Full Text Sources
Other Literature Sources