Astrocytic Modulation of Neuronal Activity in the Suprachiasmatic Nucleus: Insights from Mathematical Modeling
- PMID: 32285754
- PMCID: PMC7401727
- DOI: 10.1177/0748730420913672
Astrocytic Modulation of Neuronal Activity in the Suprachiasmatic Nucleus: Insights from Mathematical Modeling
Abstract
The suprachiasmatic nucleus (SCN) of the hypothalamus consists of a highly heterogeneous neuronal population networked together to allow precise and robust circadian timekeeping in mammals. While the critical importance of SCN neurons in regulating circadian rhythms has been extensively studied, the roles of SCN astrocytes in circadian system function are not well understood. Recent experiments have demonstrated that SCN astrocytes are circadian oscillators with the same functional clock genes as SCN neurons. Astrocytes generate rhythmic outputs that are thought to modulate neuronal activity through pre- and postsynaptic interactions. In this study, we developed an in silico multicellular model of the SCN clock to investigate the impact of astrocytes in modulating neuronal activity and affecting key clock properties such as circadian rhythmicity, period, and synchronization. The model predicted that astrocytes could alter the rhythmic activity of neurons via bidirectional interactions at tripartite synapses. Specifically, astrocyte-regulated extracellular glutamate was predicted to increase neuropeptide signaling from neurons. Consistent with experimental results, we found that astrocytes could increase the circadian period and enhance neural synchronization according to their endogenous circadian period. The impact of astrocytic modulation of circadian rhythm amplitude, period, and synchronization was predicted to be strongest when astrocytes had periods between 0 and 2 h longer than neurons. Increasing the number of neurons coupled to the astrocyte also increased its impact on period modulation and synchrony. These computational results suggest that signals that modulate astrocytic rhythms or signaling (e.g., as a function of season, age, or treatment) could cause disruptions in circadian rhythm or serve as putative therapeutic targets.
Keywords: astrocytes; circadian rhythms; mathematical modeling; neuronal coupling; suprachiasmatic nucleus.
Conflict of interest statement
CONFLICT OF INTEREST STATEMENT
The authors declare that there is no conflict of interest.
Figures
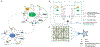
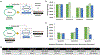
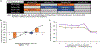
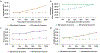
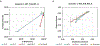
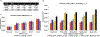
Similar articles
-
Astrocytes Control Circadian Timekeeping in the Suprachiasmatic Nucleus via Glutamatergic Signaling.Neuron. 2017 Mar 22;93(6):1420-1435.e5. doi: 10.1016/j.neuron.2017.02.030. Epub 2017 Mar 9. Neuron. 2017. PMID: 28285822 Free PMC article.
-
Cell-autonomous clock of astrocytes drives circadian behavior in mammals.Science. 2019 Jan 11;363(6423):187-192. doi: 10.1126/science.aat4104. Science. 2019. PMID: 30630934 Free PMC article.
-
Rhythmic astrocytic GABA production synchronizes neuronal circadian timekeeping in the suprachiasmatic nucleus.EMBO J. 2025 Jan;44(2):356-381. doi: 10.1038/s44318-024-00324-w. Epub 2024 Dec 2. EMBO J. 2025. PMID: 39623138 Free PMC article.
-
[Mechanisms of structural plasticity associated with photic synchronization of the circadian clock within the suprachiasmatic nucleus].J Soc Biol. 2009;203(1):49-63. doi: 10.1051/jbio:2009004. Epub 2009 Apr 10. J Soc Biol. 2009. PMID: 19358811 Review. French.
-
Cell autonomy and synchrony of suprachiasmatic nucleus circadian oscillators.Trends Neurosci. 2011 Jul;34(7):349-58. doi: 10.1016/j.tins.2011.05.003. Epub 2011 Jun 12. Trends Neurosci. 2011. PMID: 21665298 Free PMC article. Review.
Cited by
-
Circadian disruption: from mouse models to molecular mechanisms and cancer therapeutic targets.Cancer Metastasis Rev. 2023 Mar;42(1):297-322. doi: 10.1007/s10555-022-10072-0. Epub 2022 Dec 14. Cancer Metastasis Rev. 2023. PMID: 36513953 Review.
-
Modelling the functional roles of synaptic and extra-synaptic γ-aminobutyric acid receptor dynamics in circadian timekeeping.J R Soc Interface. 2021 Sep;18(182):20210454. doi: 10.1098/rsif.2021.0454. Epub 2021 Sep 15. J R Soc Interface. 2021. PMID: 34520693 Free PMC article.
-
Network Structure of the Master Clock Is Important for Its Primary Function.Front Physiol. 2021 Aug 16;12:678391. doi: 10.3389/fphys.2021.678391. eCollection 2021. Front Physiol. 2021. PMID: 34483953 Free PMC article. Review.
-
Circadian modulation of microglial physiological processes and immune responses.Glia. 2023 Feb;71(2):155-167. doi: 10.1002/glia.24261. Epub 2022 Aug 16. Glia. 2023. PMID: 35971989 Free PMC article. Review.
References
-
- Albus H, Vansteensel MJ, Michel S, Block GD, and Meijer JH (2005) A GABAergic mechanism is necessary for coupling dissociable ventral and dorsal regional oscillators within the circadian clock. Curr Biol 15:886–893. - PubMed
-
- Araque A, Parpura V, Sanzgiri RP, and Haydon PG (1999) Tripartite synapses: Glia, the unacknowledged partner. Trends Neurosci 22:208–215. - PubMed
Publication types
MeSH terms
Grants and funding
LinkOut - more resources
Full Text Sources