Advantages of Acute Brain Slices Prepared at Physiological Temperature in the Characterization of Synaptic Functions
- PMID: 32265664
- PMCID: PMC7096554
- DOI: 10.3389/fncel.2020.00063
Advantages of Acute Brain Slices Prepared at Physiological Temperature in the Characterization of Synaptic Functions
Abstract
Acute brain slice preparation is a powerful experimental model for investigating the characteristics of synaptic function in the brain. Although brain tissue is usually cut at ice-cold temperature (CT) to facilitate slicing and avoid neuronal damage, exposure to CT causes molecular and architectural changes of synapses. To address these issues, we investigated ultrastructural and electrophysiological features of synapses in mouse acute cerebellar slices prepared at ice-cold and physiological temperature (PT). In the slices prepared at CT, we found significant spine loss and reconstruction, synaptic vesicle rearrangement and decrease in synaptic proteins, all of which were not detected in slices prepared at PT. Consistent with these structural findings, slices prepared at PT showed higher release probability. Furthermore, preparation at PT allows electrophysiological recording immediately after slicing resulting in higher detectability of long-term depression (LTD) after motor learning compared with that at CT. These results indicate substantial advantages of the slice preparation at PT for investigating synaptic functions in different physiological conditions.
Keywords: acute brain slices; cerebellum; electron microscopy; patch-clamp recording; super-resolution microscopy; synaptic plasticity; synaptic transmission.
Copyright © 2020 Eguchi, Velicky, Hollergschwandtner, Itakura, Fukazawa, Danzl and Shigemoto.
Figures
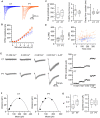
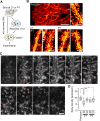
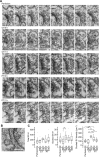
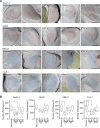
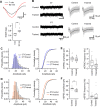
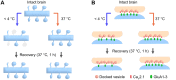
Similar articles
-
Patch-Clamp Recording of Low Frequency Stimulation-induced Long-Term Synaptic Depression in Rat Hippocampus Slices During Early and Late Neurodevelopment.Alcohol Clin Exp Res. 2021 Feb;45(2):351-364. doi: 10.1111/acer.14516. Epub 2021 Feb 2. Alcohol Clin Exp Res. 2021. PMID: 33196109
-
In vitro synaptic reconsolidation in amygdala slices prepared from rat brains.Biochem Biophys Res Commun. 2011 Apr 8;407(2):339-42. doi: 10.1016/j.bbrc.2011.03.019. Epub 2011 Mar 6. Biochem Biophys Res Commun. 2011. PMID: 21385565
-
Preparation of acute hippocampal slices from rats and transgenic mice for the study of synaptic alterations during aging and amyloid pathology.J Vis Exp. 2011 Mar 23;(49):2330. doi: 10.3791/2330. J Vis Exp. 2011. PMID: 21490565 Free PMC article.
-
Imaging of spine synapses using super-resolution microscopy.Anat Sci Int. 2021 Jun;96(3):343-358. doi: 10.1007/s12565-021-00603-0. Epub 2021 Jan 18. Anat Sci Int. 2021. PMID: 33459976 Review.
-
Learning-induced structural plasticity in the cerebellum.Int Rev Neurobiol. 2014;117:1-19. doi: 10.1016/B978-0-12-420247-4.00001-4. Int Rev Neurobiol. 2014. PMID: 25172626 Review.
Cited by
-
Nanoscale Phosphoinositide Distribution on Cell Membranes of Mouse Cerebellar Neurons.J Neurosci. 2023 Jun 7;43(23):4197-4216. doi: 10.1523/JNEUROSCI.1514-22.2023. Epub 2023 May 9. J Neurosci. 2023. PMID: 37160366 Free PMC article.
-
Prioritized docking of synaptic vesicles provided by a rapid recycling pathway.iScience. 2023 Mar 9;26(4):106366. doi: 10.1016/j.isci.2023.106366. eCollection 2023 Apr 21. iScience. 2023. PMID: 37009220 Free PMC article.
-
Putative neurochemical and cell type contributions to hemodynamic activity in the rodent caudate putamen.J Cereb Blood Flow Metab. 2023 Apr;43(4):481-498. doi: 10.1177/0271678X221142533. Epub 2022 Nov 30. J Cereb Blood Flow Metab. 2023. PMID: 36448509 Free PMC article. Review.
-
13C Isotope Labeling and Mass Spectrometric Isotope Enrichment Analysis in Acute Brain Slices.Methods Mol Biol. 2023;2675:181-194. doi: 10.1007/978-1-0716-3247-5_14. Methods Mol Biol. 2023. PMID: 37258764
-
Ultrastructural Imaging of Activity-Dependent Synaptic Membrane-Trafficking Events in Cultured Brain Slices.Neuron. 2020 Dec 9;108(5):843-860.e8. doi: 10.1016/j.neuron.2020.09.004. Epub 2020 Sep 28. Neuron. 2020. PMID: 32991831 Free PMC article.
References
Grants and funding
LinkOut - more resources
Full Text Sources