Double-Stranded-RNA-Binding Protein 2 Participates in Antiviral Defense
- PMID: 32213615
- PMCID: PMC7269452
- DOI: 10.1128/JVI.00017-20
Double-Stranded-RNA-Binding Protein 2 Participates in Antiviral Defense
Abstract
Double-stranded RNA (dsRNA) is a common pattern formed during the replication of both RNA and DNA viruses. Perception of virus-derived dsRNAs by specialized receptor molecules leads to the activation of various antiviral measures. In plants, these defensive processes include the adaptive RNA interference (RNAi) pathway and innate pattern-triggered immune (PTI) responses. While details of the former process have been well established in recent years, the latter are still only partially understood at the molecular level. Nonetheless, emerging data suggest extensive cross talk between the different antiviral mechanisms. Here, we demonstrate that dsRNA-binding protein 2 (DRB2) of Nicotiana benthamiana plays a direct role in potato virus X (PVX)-elicited systemic necrosis. These results establish that DRB2, a known component of RNAi, is also involved in a virus-induced PTI response. In addition, our findings suggest that RNA-dependent polymerase 6 (RDR6)-dependent dsRNAs play an important role in the triggering of PVX-induced systemic necrosis. Based on our data, a model is formulated whereby competition between different DRB proteins for virus-derived dsRNAs helps establish the dominant antiviral pathways that are activated in response to virus infection.IMPORTANCE Plants employ multiple defense mechanisms to restrict viral infections, among which RNA interference is the best understood. The activation of innate immunity often leads to both local and systemic necrotic responses, which confine the virus to the infected cells and can also provide resistance to distal, noninfected parts of the organism. Systemic necrosis, which is regarded as a special form of the local hypersensitive response, results in necrosis of the apical stem region, usually causing the death of the plant. Here, we provide evidence that the dsRNA-binding protein 2 of Nicotiana benthamiana plays an important role in virus-induced systemic necrosis. Our findings are not only compatible with the recent hypothesis that DRB proteins act as viral invasion sensors but also extends it by proposing that DRBs play a critical role in establishing the dominant antiviral measures that are triggered during virus infection.
Keywords: DRB2; PAMP-triggered immunity; RDR6; antiviral RNA interference; double-stranded RNA.
Copyright © 2020 American Society for Microbiology.
Figures
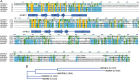
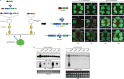
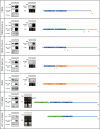
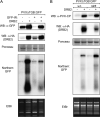
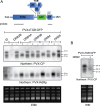
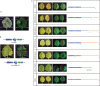
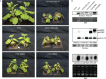
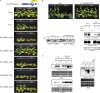
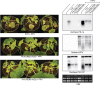
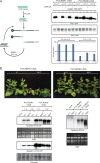
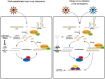
Similar articles
-
RNA silencing-related genes contribute to tolerance of infection with potato virus X and Y in a susceptible tomato plant.Virol J. 2020 Oct 8;17(1):149. doi: 10.1186/s12985-020-01414-x. Virol J. 2020. PMID: 33032637 Free PMC article.
-
Comparative analysis of RNA interference and pattern-triggered immunity induced by dsRNA reveals different efficiencies in the antiviral response to potato virus X.Mol Plant Pathol. 2024 Sep;25(9):e70008. doi: 10.1111/mpp.70008. Mol Plant Pathol. 2024. PMID: 39290152 Free PMC article.
-
Double-stranded RNAs induce a pattern-triggered immune signaling pathway in plants.New Phytol. 2016 Aug;211(3):1008-19. doi: 10.1111/nph.13944. Epub 2016 Mar 31. New Phytol. 2016. PMID: 27030513
-
Perception of double-stranded RNA in plant antiviral immunity.Mol Plant Pathol. 2019 Sep;20(9):1203-1210. doi: 10.1111/mpp.12798. Epub 2019 Apr 3. Mol Plant Pathol. 2019. PMID: 30942534 Free PMC article. Review.
-
Nucleic acid-mediated PAMP-triggered immunity in plants.Curr Opin Virol. 2020 Jun;42:32-39. doi: 10.1016/j.coviro.2020.04.003. Epub 2020 Jun 3. Curr Opin Virol. 2020. PMID: 32504993 Review.
Cited by
-
RNA silencing-related genes contribute to tolerance of infection with potato virus X and Y in a susceptible tomato plant.Virol J. 2020 Oct 8;17(1):149. doi: 10.1186/s12985-020-01414-x. Virol J. 2020. PMID: 33032637 Free PMC article.
-
RBM39 Alters Phosphorylation of c-Jun and Binds to Viral RNA to Promote PRRSV Proliferation.Front Immunol. 2021 May 17;12:664417. doi: 10.3389/fimmu.2021.664417. eCollection 2021. Front Immunol. 2021. PMID: 34079549 Free PMC article.
-
Bioinformatic Analysis Predicts a Novel Genetic Module Related to Triple Gene and Binary Movement Blocks of Plant Viruses: Tetra-Cistron Movement Block.Biomolecules. 2022 Jun 21;12(7):861. doi: 10.3390/biom12070861. Biomolecules. 2022. PMID: 35883420 Free PMC article.
-
A Review of Vector-Borne Rice Viruses.Viruses. 2022 Oct 14;14(10):2258. doi: 10.3390/v14102258. Viruses. 2022. PMID: 36298813 Free PMC article. Review.
-
dsRNA-induced immunity targets plasmodesmata and is suppressed by viral movement proteins.Plant Cell. 2023 Sep 27;35(10):3845-3869. doi: 10.1093/plcell/koad176. Plant Cell. 2023. PMID: 37378592 Free PMC article.
References
Publication types
MeSH terms
Substances
LinkOut - more resources
Full Text Sources