Cell Stress Induced Stressome Release Including Damaged Membrane Vesicles and Extracellular HSP90 by Prostate Cancer Cells
- PMID: 32204513
- PMCID: PMC7140686
- DOI: 10.3390/cells9030755
Cell Stress Induced Stressome Release Including Damaged Membrane Vesicles and Extracellular HSP90 by Prostate Cancer Cells
Abstract
Tumor cells exhibit therapeutic stress resistance-associated secretory phenotype involving extracellular vesicles (EVs) such as oncosomes and heat shock proteins (HSPs). Such a secretory phenotype occurs in response to cell stress and cancer therapeutics. HSPs are stress-responsive molecular chaperones promoting proper protein folding, while also being released from cells with EVs as well as a soluble form known as alarmins. We have here investigated the secretory phenotype of castration-resistant prostate cancer (CRPC) cells using proteome analysis. We have also examined the roles of the key co-chaperone CDC37 in the release of EV proteins including CD9 and epithelial-to-mesenchymal transition (EMT), a key event in tumor progression. EVs derived from CRPC cells promoted EMT in normal prostate epithelial cells. Some HSP family members and their potential receptor CD91/LRP1 were enriched at high levels in CRPC cell-derived EVs among over 700 other protein types found by mass spectrometry. The small EVs (30-200 nm in size) were released even in a non-heated condition from the prostate cancer cells, whereas the EMT-coupled release of EVs (200-500 nm) and damaged membrane vesicles with associated HSP90α was increased after heat shock stress (HSS). GAPDH and lactate dehydrogenase, a marker of membrane leakage/damage, were also found in conditioned media upon HSS. During this stress response, the intracellular chaperone CDC37 was transcriptionally induced by heat shock factor 1 (HSF1), which activated the CDC37 core promoter, containing an interspecies conserved heat shock element. In contrast, knockdown of CDC37 decreased EMT-coupled release of CD9-containing vesicles. Triple siRNA targeting CDC37, HSP90α, and HSP90β was required for efficient reduction of this chaperone trio and to reduce tumorigenicity of the CRPC cells in vivo. Taken together, we define "stressome" as cellular stress-induced all secretion products, including EVs (200-500 nm), membrane-damaged vesicles and remnants, and extracellular HSP90 and GAPDH. Our data also indicated that CDC37 is crucial for the release of vesicular proteins and tumor progression in prostate cancer.
Keywords: cell division control 37 (CDC37); cell stress response; ectosome; exosome; extracellular vesicle; heat shock protein 90 (HSP90); prostate cancer; stressome.
Conflict of interest statement
The authors declare no conflict of interest.
Figures
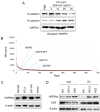
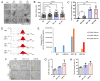
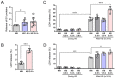
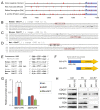
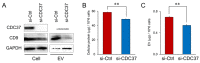
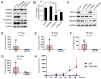
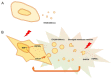
Similar articles
-
Triple knockdown of CDC37, HSP90-alpha and HSP90-beta diminishes extracellular vesicles-driven malignancy events and macrophage M2 polarization in oral cancer.J Extracell Vesicles. 2020 May 31;9(1):1769373. doi: 10.1080/20013078.2020.1769373. J Extracell Vesicles. 2020. PMID: 33144925 Free PMC article.
-
Suppression of heat shock protein 27 using OGX-427 induces endoplasmic reticulum stress and potentiates heat shock protein 90 inhibitors to delay castrate-resistant prostate cancer.Eur Urol. 2014 Jul;66(1):145-55. doi: 10.1016/j.eururo.2013.12.019. Epub 2013 Dec 29. Eur Urol. 2014. PMID: 24411988 Free PMC article.
-
HSP-enriched properties of extracellular vesicles involve survival of metastatic oral cancer cells.J Cell Biochem. 2018 Sep;119(9):7350-7362. doi: 10.1002/jcb.27039. Epub 2018 May 16. J Cell Biochem. 2018. PMID: 29768689
-
Roles of Extracellular HSPs as Biomarkers in Immune Surveillance and Immune Evasion.Int J Mol Sci. 2019 Sep 17;20(18):4588. doi: 10.3390/ijms20184588. Int J Mol Sci. 2019. PMID: 31533245 Free PMC article. Review.
-
Cdc37 as a co-chaperone to Hsp90.Subcell Biochem. 2015;78:103-12. doi: 10.1007/978-3-319-11731-7_5. Subcell Biochem. 2015. PMID: 25487018 Free PMC article. Review.
Cited by
-
Role of Extracellular Vesicles in Compromising Cellular Resilience to Environmental Stressors.Biomed Res Int. 2021 Jul 20;2021:9912281. doi: 10.1155/2021/9912281. eCollection 2021. Biomed Res Int. 2021. PMID: 34337063 Free PMC article. Review.
-
Triple knockdown of CDC37, HSP90-alpha and HSP90-beta diminishes extracellular vesicles-driven malignancy events and macrophage M2 polarization in oral cancer.J Extracell Vesicles. 2020 May 31;9(1):1769373. doi: 10.1080/20013078.2020.1769373. J Extracell Vesicles. 2020. PMID: 33144925 Free PMC article.
-
Dairy: Friend or Foe? Bovine Milk-Derived Extracellular Vesicles and Autoimmune Diseases.Int J Mol Sci. 2024 Oct 26;25(21):11499. doi: 10.3390/ijms252111499. Int J Mol Sci. 2024. PMID: 39519052 Free PMC article. Review.
-
Hypoxia-Regulated Tumor-Derived Exosomes and Tumor Progression: A Focus on Immune Evasion.Int J Mol Sci. 2022 Oct 4;23(19):11789. doi: 10.3390/ijms231911789. Int J Mol Sci. 2022. PMID: 36233088 Free PMC article. Review.
-
Tumor cell-derived exosomes regulate macrophage polarization: Emerging directions in the study of tumor genesis and development.Heliyon. 2023 Aug 22;9(9):e19296. doi: 10.1016/j.heliyon.2023.e19296. eCollection 2023 Sep. Heliyon. 2023. PMID: 37662730 Free PMC article. Review.
References
-
- Namba Y., Sogawa C., Okusha Y., Kawai H., Itagaki M., Ono K., Murakami J., Aoyama E., Ohyama K., Asaumi J.-I., et al. Depletion of Lipid Efflux Pump ABCG1 Triggers the Intracellular Accumulation of Extracellular Vesicles and Reduces Aggregation and Tumorigenesis of Metastatic Cancer Cells. Front. Oncol. 2018;8:376. doi: 10.3389/fonc.2018.00376. - DOI - PMC - PubMed
-
- Dias T.R., Samanta L., Agarwal A., Pushparaj P.N., Selvam M.P., Sharma R. Proteomic Signatures Reveal Differences in Stress Response, Antioxidant Defense and Proteasomal Activity in Fertile Men with High Seminal ROS Levels. Int. J. Mol. Sci. 2019;20:203. doi: 10.3390/ijms20010203. - DOI - PMC - PubMed
Publication types
MeSH terms
Substances
Grants and funding
LinkOut - more resources
Full Text Sources
Medical
Research Materials
Miscellaneous