Breaking the vicious loop between inflammation, oxidative stress and coagulation, a novel anti-thrombus insight of nattokinase by inhibiting LPS-induced inflammation and oxidative stress
- PMID: 32193146
- PMCID: PMC7078552
- DOI: 10.1016/j.redox.2020.101500
Breaking the vicious loop between inflammation, oxidative stress and coagulation, a novel anti-thrombus insight of nattokinase by inhibiting LPS-induced inflammation and oxidative stress
Abstract
Thrombosis is a principle cause of cardiovascular disease, the leading cause of morbidity and mortality worldwide; however, the conventional anti-thrombotic approach often leads to bleeding complications despite extensive clinical management and monitoring. In view of the intense crosstalk between inflammation and coagulation, plus the contributing role of ROS to both inflammation and coagulation, it is highly desirable to develop safer anti-thrombotic agent with preserved anti-inflammatory and anti-oxidative stress activities. Nattokinase (NK) possesses many beneficial effects on cardiovascular system due to its strong thrombolytic and anticoagulant activities. Herein, we demonstrated that NK not only effectively prevented xylene-induced ear oedema in mice, but also remarkably protected against LPS-induced acute kidney injury in mice through restraining inflammation and oxidative stress, a central player in the initiation and progression of inflammation. Fascinatingly, in line with our in vivo data, NK elicited prominent anti-inflammatory activity in RAW264.7 macrophages via suppressing the LPS-induced TLR4 and NOX2 activation, thereby repressing the corresponding ROS production, MAPKs activation, and NF-κB translocation from the cytoplasm to the nucleus, where it mediates the expression of pro-inflammatory mediators, such as TNF-α, IL-6, NO, and PAI-1 in activated macrophage cells. In particular, consistent with the macrophage studies, NK markedly inhibited serum PAI-1 levels induced by LPS, thereby blocking the deposition of fibrin in the glomeruli of endotoxin-treated animals. In summary, we extended the anti-thrombus mechanism of NK by demonstrating the anti-inflammatory and anti-oxidative stress effects of NK in ameliorating LPS-activated macrophage signaling and protecting against LPS-stimulated AKI as well as glomeruler thrombus in mice, opening a comprehensive anti-thrombus strategy by breaking the vicious cycle between inflammation, oxidative stress and thrombosis.
Keywords: Inflammation; NOX2; Nattokinase; Oxidative stress; TRL4; Thrombus.
Copyright © 2020 The Authors. Published by Elsevier B.V. All rights reserved.
Conflict of interest statement
Declaration of competing interest There is no conflict of interests.
Figures
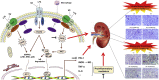
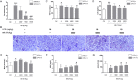
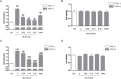
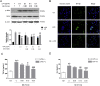
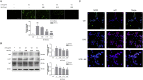
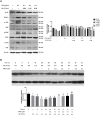
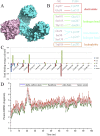
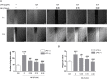
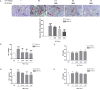
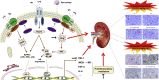
Similar articles
-
Inhibition of HDAC6 attenuates LPS-induced inflammation in macrophages by regulating oxidative stress and suppressing the TLR4-MAPK/NF-κB pathways.Biomed Pharmacother. 2019 Sep;117:109166. doi: 10.1016/j.biopha.2019.109166. Epub 2019 Jun 27. Biomed Pharmacother. 2019. PMID: 31255993
-
Aloperine suppresses LPS-induced macrophage activation through inhibiting the TLR4/NF-κB pathway.Inflamm Res. 2020 Apr;69(4):375-383. doi: 10.1007/s00011-019-01313-0. Epub 2020 Mar 7. Inflamm Res. 2020. PMID: 32144444
-
Anti-inflammatory effects of Aureusidin in LPS-stimulated RAW264.7 macrophages via suppressing NF-κB and activating ROS- and MAPKs-dependent Nrf2/HO-1 signaling pathways.Toxicol Appl Pharmacol. 2020 Jan 15;387:114846. doi: 10.1016/j.taap.2019.114846. Epub 2019 Nov 29. Toxicol Appl Pharmacol. 2020. PMID: 31790703
-
Nattokinase as an adjuvant therapeutic strategy for non-communicable diseases: a review of fibrinolytic, antithrombotic, anti-inflammatory, and antioxidant effects.Expert Rev Cardiovasc Ther. 2024 Oct;22(10):565-574. doi: 10.1080/14779072.2024.2416663. Epub 2024 Oct 15. Expert Rev Cardiovasc Ther. 2024. PMID: 39404094 Review.
-
Microbial nattokinase: from synthesis to potential application.Food Funct. 2023 Mar 20;14(6):2568-2585. doi: 10.1039/d2fo03389e. Food Funct. 2023. PMID: 36857725 Review.
Cited by
-
Evaluation of Antioxidant Defence Systems and Inflammatory Status in Basketball Elite Athletes.Genes (Basel). 2023 Sep 29;14(10):1891. doi: 10.3390/genes14101891. Genes (Basel). 2023. PMID: 37895240 Free PMC article.
-
Data Recorded in Real Life Support the Safety of Nattokinase in Patients with Vascular Diseases.Nutrients. 2021 Jun 13;13(6):2031. doi: 10.3390/nu13062031. Nutrients. 2021. PMID: 34199189 Free PMC article. Clinical Trial.
-
Effect of nattokinase on the pathological conditions in streptozotocin induced diabetic rats.Heliyon. 2024 Mar 27;10(7):e28835. doi: 10.1016/j.heliyon.2024.e28835. eCollection 2024 Apr 15. Heliyon. 2024. PMID: 38586318 Free PMC article.
-
Bibliometric and visual analysis of immunisation associated with acute kidney injury from 2003 to 2023.Front Pharmacol. 2024 Jul 1;15:1388527. doi: 10.3389/fphar.2024.1388527. eCollection 2024. Front Pharmacol. 2024. PMID: 39011500 Free PMC article.
-
Evaluation of organ function in patients with severe COVID-19 infections.Med Clin (Barc). 2020 Sep 11;155(5):191-196. doi: 10.1016/j.medcli.2020.05.012. Epub 2020 Jun 4. Med Clin (Barc). 2020. PMID: 32586669 Free PMC article.
References
-
- Ouriel K., Welch E.L., Shortell C.K., Geary K., Fiore W.M., Cimino C. Comparison of streptokinase, urokinase, and recombinant tissue plasminogen activator in an in vitro model of venous thrombolysis. J. Vasc. Surg. 1995;22(5):593–597. - PubMed
-
- Raffort J., Lareyre F., Clement M., Hassen-Khodja R., Chinetti G., MallatZ Monocytes and macrophages in abdominal aortic aneurysm. Nat. Rev. Cardiol. 2017;14:457–471. - PubMed
-
- Levi M., van der Poll T., Büller H.R. Bidirectional relation between inflammation and coagulation. Circulation. 2004;109(22):2698–2704. - PubMed
-
- Jackson S.P., Darbousset R., Schoenwaelder S.M. Thromboinflammation: challenges of therapeutically targeting coagulation and other host defense mechanisms. Blood. 2019;133:906–918. - PubMed
Publication types
MeSH terms
Substances
LinkOut - more resources
Full Text Sources
Medical
Miscellaneous