Structure-Guided Mutagenesis Alters Deubiquitinating Activity and Attenuates Pathogenesis of a Murine Coronavirus
- PMID: 32188728
- PMCID: PMC7269429
- DOI: 10.1128/JVI.01734-19
Structure-Guided Mutagenesis Alters Deubiquitinating Activity and Attenuates Pathogenesis of a Murine Coronavirus
Abstract
Coronaviruses express a multifunctional papain-like protease, termed papain-like protease 2 (PLP2). PLP2 acts as a protease that cleaves the viral replicase polyprotein and as a deubiquitinating (DUB) enzyme which removes ubiquitin (Ub) moieties from ubiquitin-conjugated proteins. Previous in vitro studies implicated PLP2/DUB activity as a negative regulator of the host interferon (IFN) response, but the role of DUB activity during virus infection was unknown. Here, we used X-ray structure-guided mutagenesis and functional studies to identify amino acid substitutions within the ubiquitin-binding surface of PLP2 that reduced DUB activity without affecting polyprotein processing activity. We engineered a DUB mutation (Asp1772 to Ala) into a murine coronavirus and evaluated the replication and pathogenesis of the DUB mutant virus (DUBmut) in cultured macrophages and in mice. We found that the DUBmut virus replicates similarly to the wild-type (WT) virus in cultured cells, but the DUBmut virus activates an IFN response at earlier times compared to the wild-type virus infection in macrophages, consistent with DUB activity negatively regulating the IFN response. We compared the pathogenesis of the DUBmut virus to that of the wild-type virus and found that the DUBmut-infected mice had a statistically significant reduction (P < 0.05) in viral titer in liver and spleen at day 5 postinfection (d p.i.), although both wild-type and DUBmut virus infections resulted in similar liver pathology. Overall, this study demonstrates that structure-guided mutagenesis aids the identification of critical determinants of the PLP2-ubiquitin complex and that PLP2/DUB activity plays a role as an interferon antagonist in coronavirus pathogenesis.IMPORTANCE Coronaviruses employ a genetic economy by encoding multifunctional proteins that function in viral replication and also modify the host environment to disarm the innate immune response. The coronavirus papain-like protease 2 (PLP2) domain possesses protease activity, which cleaves the viral replicase polyprotein, and also DUB activity (deconjugating ubiquitin/ubiquitin-like molecules from modified substrates) using identical catalytic residues. To separate the DUB activity from the protease activity, we employed a structure-guided mutagenesis approach and identified residues that are important for ubiquitin binding. We found that mutating the ubiquitin-binding residues results in a PLP2 that has reduced DUB activity but retains protease activity. We engineered a recombinant murine coronavirus to express the DUB mutant and showed that the DUB mutant virus activated an earlier type I interferon response in macrophages and exhibited reduced replication in mice. The results of this study demonstrate that PLP2/DUB is an interferon antagonist and a virulence trait of coronaviruses.
Keywords: DUB activity; IFN antagonist; PLP2; PLP2 structure; coronavirus; papain-like protease.
Copyright © 2020 American Society for Microbiology.
Figures
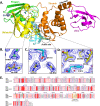
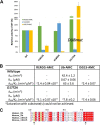
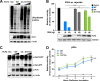
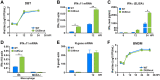
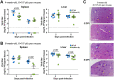
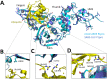
Similar articles
-
Coronavirus Endoribonuclease and Deubiquitinating Interferon Antagonists Differentially Modulate the Host Response during Replication in Macrophages.J Virol. 2020 May 18;94(11):e00178-20. doi: 10.1128/JVI.00178-20. Print 2020 May 18. J Virol. 2020. PMID: 32188729 Free PMC article.
-
Deubiquitinase function of arterivirus papain-like protease 2 suppresses the innate immune response in infected host cells.Proc Natl Acad Sci U S A. 2013 Feb 26;110(9):E838-47. doi: 10.1073/pnas.1218464110. Epub 2013 Feb 11. Proc Natl Acad Sci U S A. 2013. PMID: 23401522 Free PMC article.
-
Murine coronavirus ubiquitin-like domain is important for papain-like protease stability and viral pathogenesis.J Virol. 2015 May;89(9):4907-17. doi: 10.1128/JVI.00338-15. Epub 2015 Feb 18. J Virol. 2015. PMID: 25694594 Free PMC article.
-
Nidovirus papain-like proteases: multifunctional enzymes with protease, deubiquitinating and deISGylating activities.Virus Res. 2014 Dec 19;194:184-90. doi: 10.1016/j.virusres.2014.01.025. Epub 2014 Feb 7. Virus Res. 2014. PMID: 24512893 Free PMC article. Review.
-
Coronaviral PLpro proteases and the immunomodulatory roles of conjugated versus free Interferon Stimulated Gene product-15 (ISG15).Semin Cell Dev Biol. 2022 Dec;132:16-26. doi: 10.1016/j.semcdb.2022.06.005. Epub 2022 Jun 25. Semin Cell Dev Biol. 2022. PMID: 35764457 Free PMC article. Review.
Cited by
-
A computational essential dynamics approach to investigate structural influences of ligand binding on Papain like protease from SARS-CoV-2.Comput Biol Chem. 2022 Aug;99:107721. doi: 10.1016/j.compbiolchem.2022.107721. Epub 2022 Jun 28. Comput Biol Chem. 2022. PMID: 35835027 Free PMC article.
-
Deubiquitinating Enzymes in Coronaviruses and Possible Therapeutic Opportunities for COVID-19.Int J Mol Sci. 2020 May 15;21(10):3492. doi: 10.3390/ijms21103492. Int J Mol Sci. 2020. PMID: 32429099 Free PMC article. Review.
-
P53 in the impaired lungs.DNA Repair (Amst). 2020 Nov;95:102952. doi: 10.1016/j.dnarep.2020.102952. Epub 2020 Aug 19. DNA Repair (Amst). 2020. PMID: 32846356 Free PMC article. Review.
-
Bioinformatics-based investigation on the genetic influence between SARS-CoV-2 infections and idiopathic pulmonary fibrosis (IPF) diseases, and drug repurposing.Sci Rep. 2023 Mar 22;13(1):4685. doi: 10.1038/s41598-023-31276-6. Sci Rep. 2023. PMID: 36949176 Free PMC article.
-
Interferon Control of Human Coronavirus Infection and Viral Evasion: Mechanistic Insights and Implications for Antiviral Drug and Vaccine Development.J Mol Biol. 2022 Mar 30;434(6):167438. doi: 10.1016/j.jmb.2021.167438. Epub 2022 Jan 3. J Mol Biol. 2022. PMID: 34990653 Free PMC article. Review.
References
-
- Niemeyer D, Mösbauer K, Klein EM, Sieberg A, Mettelman RC, Mielech AM, Dijkman R, Baker SC, Drosten C, Müller MA. 2018. The papain-like protease determines a virulence trait that varies among members of the SARS-coronavirus species. PLoS Pathog 14:e1007296. doi:10.1371/journal.ppat.1007296. - DOI - PMC - PubMed
Publication types
MeSH terms
Substances
Grants and funding
LinkOut - more resources
Full Text Sources