Stochastic activation and bistability in a Rab GTPase regulatory network
- PMID: 32161136
- PMCID: PMC7104049
- DOI: 10.1073/pnas.1921027117
Stochastic activation and bistability in a Rab GTPase regulatory network
Abstract
The eukaryotic endomembrane system is controlled by small GTPases of the Rab family, which are activated at defined times and locations in a switch-like manner. While this switch is well understood for an individual protein, how regulatory networks produce intracellular activity patterns is currently not known. Here, we combine in vitro reconstitution experiments with computational modeling to study a minimal Rab5 activation network. We find that the molecular interactions in this system give rise to a positive feedback and bistable collective switching of Rab5. Furthermore, we find that switching near the critical point is intrinsically stochastic and provide evidence that controlling the inactive population of Rab5 on the membrane can shape the network response. Notably, we demonstrate that collective switching can spread on the membrane surface as a traveling wave of Rab5 activation. Together, our findings reveal how biochemical signaling networks control vesicle trafficking pathways and how their nonequilibrium properties define the spatiotemporal organization of the cell.
Keywords: Rab5; bistability; positive feedback; stochasticity.
Conflict of interest statement
The authors declare no competing interest.
Figures
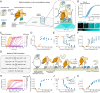
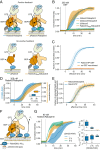
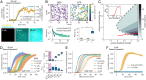
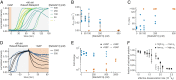
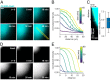
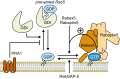
Similar articles
-
Delayed onset of positive feedback activation of Rab5 by Rabex-5 and Rabaptin-5 in endocytosis.PLoS One. 2010 Feb 16;5(2):e9226. doi: 10.1371/journal.pone.0009226. PLoS One. 2010. PMID: 20169068 Free PMC article.
-
Structure and Dynamics of Mono- vs. Doubly Lipidated Rab5 in Membranes.Int J Mol Sci. 2019 Sep 26;20(19):4773. doi: 10.3390/ijms20194773. Int J Mol Sci. 2019. PMID: 31561436 Free PMC article.
-
A novel binding protein composed of homophilic tetramer exhibits unique properties for the small GTPase Rab5.J Biol Chem. 2002 Feb 1;277(5):3412-8. doi: 10.1074/jbc.M106276200. Epub 2001 Dec 3. J Biol Chem. 2002. PMID: 11733506
-
Membrane recruitment of effector proteins by Arf and Rab GTPases.Curr Opin Struct Biol. 2005 Dec;15(6):681-9. doi: 10.1016/j.sbi.2005.10.015. Epub 2005 Nov 9. Curr Opin Struct Biol. 2005. PMID: 16289847 Review.
-
Biogenesis of the sorting endosome: the role of Rab5.Traffic. 2000 Sep;1(9):695-701. doi: 10.1034/j.1600-0854.2000.010902.x. Traffic. 2000. PMID: 11208157 Review.
Cited by
-
Design principles of improving the dose-response alignment in coupled GTPase switches.NPJ Syst Biol Appl. 2023 Jan 31;9(1):3. doi: 10.1038/s41540-023-00266-9. NPJ Syst Biol Appl. 2023. PMID: 36720885 Free PMC article.
-
Targeting of the Mon1-Ccz1 Rab guanine nucleotide exchange factor to distinct organelles by a synergistic protein and lipid code.J Biol Chem. 2023 Mar;299(3):102915. doi: 10.1016/j.jbc.2023.102915. Epub 2023 Jan 14. J Biol Chem. 2023. PMID: 36649906 Free PMC article.
-
Stochasticity and positive feedback enable enzyme kinetics at the membrane to sense reaction size.Proc Natl Acad Sci U S A. 2021 Nov 23;118(47):e2103626118. doi: 10.1073/pnas.2103626118. Proc Natl Acad Sci U S A. 2021. PMID: 34789575 Free PMC article.
-
A novel partitivirus conferring hypovirulence by affecting vesicle transport in the fungus Colletotrichum.mBio. 2024 Feb 14;15(2):e0253023. doi: 10.1128/mbio.02530-23. Epub 2024 Jan 9. mBio. 2024. PMID: 38193704 Free PMC article.
-
Impact of C-terminal truncations in the Arabidopsis Rab escort protein (REP) on REP-Rab interaction and plant fertility.Plant J. 2021 Dec;108(5):1400-1421. doi: 10.1111/tpj.15519. Epub 2021 Oct 16. Plant J. 2021. PMID: 34592024 Free PMC article.
References
-
- Pomerening J. R., Sontag E. D., Ferrell J. E. Jr, Building a cell cycle oscillator: Hysteresis and bistability in the activation of Cdc2. Nat. Cell Biol. 5, 346–351 (2003). - PubMed
-
- Gardner T. S., Cantor C. R., Collins J. J., Construction of a genetic toggle switch in Escherichia coli. Nature 403, 339–342 (2000). - PubMed
Publication types
MeSH terms
Substances
Grants and funding
LinkOut - more resources
Full Text Sources