Zinc modulation of proton currents in a new voltage-gated proton channel suggests a mechanism of inhibition
- PMID: 32160407
- PMCID: PMC7754295
- DOI: 10.1111/febs.15291
Zinc modulation of proton currents in a new voltage-gated proton channel suggests a mechanism of inhibition
Abstract
The HV 1 voltage-gated proton (HV 1) channel is a key component of the cellular proton extrusion machinery and is pivotal for charge compensation during the respiratory burst of phagocytes. The best-described physiological inhibitor of HV 1 is Zn2+ . Externally applied ZnCl2 drastically reduces proton currents reportedly recorded in Homo sapiens, Rattus norvegicus, Mus musculus, Oryctolagus cuniculus, Rana esculenta, Helix aspersa, Ciona intestinalis, Coccolithus pelagicus, Emiliania huxleyi, Danio rerio, Helisoma trivolvis, and Lingulodinium polyedrum, but with considerable species variability. Here, we report the effects of Zn2+ and Cd2+ on HV 1 from Nicoletia phytophila, NpHV 1. We introduced mutations at potential Zn2+ coordination sites and measured Zn2+ inhibition in different extracellular pH, with Zn2+ concentrations up to 1000 μm. Zn2+ inhibition in NpHV 1 was quantified by the slowing of the activation time constant and a positive shift of the conductance-voltage curve. Replacing aspartate in the S3-S4 loop with histidine (D145H) enhanced both the slowing of activation kinetics and the shift in the voltage-conductance curve, such that Zn2+ inhibition closely resembled that of the human channel. Histidine is much more effective than aspartate in coordinating Zn2+ in the S3-S4 linker. A simple Hodgkin Huxley model of NpHV 1 suggests a decrease in the opening rate if it is inhibited by zinc or cadmium. Limiting slope measurements and high-resolution clear native gel electrophoresis (hrCNE) confirmed that NpHV 1 functions as a dimer. The data support the hypothesis that zinc is coordinated in between the dimer instead of the monomer. Zinc coordination sites may be potential targets for drug development.
Keywords: HV1; ion channel; patch-clamp; structure-function; zinc.
© 2020 The Authors. The FEBS Journal published by John Wiley & Sons Ltd on behalf of Federation of European Biochemical Societies.
Conflict of interest statement
The authors declare no conflict of interest.
Figures
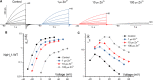
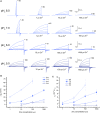
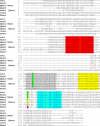
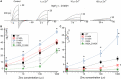
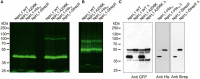
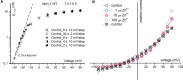
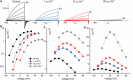
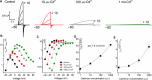
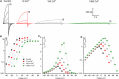
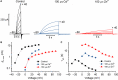
Similar articles
-
Exotic properties of a voltage-gated proton channel from the snail Helisoma trivolvis.J Gen Physiol. 2018 Jun 4;150(6):835-850. doi: 10.1085/jgp.201711967. Epub 2018 May 9. J Gen Physiol. 2018. PMID: 29743301 Free PMC article.
-
Identification of an HV 1 voltage-gated proton channel in insects.FEBS J. 2016 Apr;283(8):1453-64. doi: 10.1111/febs.13680. Epub 2016 Mar 6. FEBS J. 2016. PMID: 26866814
-
pH-dependent inhibition of voltage-gated H(+) currents in rat alveolar epithelial cells by Zn(2+) and other divalent cations.J Gen Physiol. 1999 Dec;114(6):819-38. doi: 10.1085/jgp.114.6.819. J Gen Physiol. 1999. PMID: 10578017 Free PMC article.
-
Pharmacology of voltage-gated proton channels.Curr Pharm Des. 2007;13(23):2400-20. doi: 10.2174/138161207781368675. Curr Pharm Des. 2007. PMID: 17692009 Review.
-
Voltage-gated proton channels.Compr Physiol. 2012 Apr;2(2):1355-85. doi: 10.1002/cphy.c100071. Compr Physiol. 2012. PMID: 23798303 Free PMC article. Review.
Cited by
-
Voltage-gated proton channels in polyneopteran insects.FEBS Open Bio. 2022 Feb;12(2):523-537. doi: 10.1002/2211-5463.13361. Epub 2022 Jan 19. FEBS Open Bio. 2022. PMID: 34986517 Free PMC article.
-
Exotic properties of a voltage-gated proton channel from the snail Helisoma trivolvis.J Gen Physiol. 2018 Jun 4;150(6):835-850. doi: 10.1085/jgp.201711967. Epub 2018 May 9. J Gen Physiol. 2018. PMID: 29743301 Free PMC article.
-
pH-Channeling in Cancer: How pH-Dependence of Cation Channels Shapes Cancer Pathophysiology.Cancers (Basel). 2020 Sep 2;12(9):2484. doi: 10.3390/cancers12092484. Cancers (Basel). 2020. PMID: 32887220 Free PMC article. Review.
-
Voltage-Gated Proton Channels in the Tree of Life.Biomolecules. 2023 Jun 24;13(7):1035. doi: 10.3390/biom13071035. Biomolecules. 2023. PMID: 37509071 Free PMC article. Review.
-
Identification of a Novel Structural Class of HV1 Inhibitors by Structure-Based Virtual Screening.J Chem Inf Model. 2024 Jun 24;64(12):4850-4862. doi: 10.1021/acs.jcim.4c00240. Epub 2024 Jun 8. J Chem Inf Model. 2024. PMID: 38850237 Free PMC article.
References
-
- Thomas RC & Meech RW (1982) Hydrogen ion currents and intracellular pH in depolarized voltage‐clamped snail neurones. Nature 299, 826–828. - PubMed
-
- Sasaki M, Takagi M & Okamura Y (2006) A voltage sensor‐domain protein is a voltage‐gated proton channel. Science 312, 589–592. - PubMed
-
- Chaves G, Derst C, Franzen A, Mashimo Y, Machida R & Musset B (2016) Identification of an HV 1 voltage‐gated proton channel in insects. FEBS J 283, 1453–464. - PubMed
Publication types
MeSH terms
Substances
LinkOut - more resources
Full Text Sources