Bacterial Defense Systems against the Neutrophilic Oxidant Hypochlorous Acid
- PMID: 32152198
- PMCID: PMC7309615
- DOI: 10.1128/IAI.00964-19
Bacterial Defense Systems against the Neutrophilic Oxidant Hypochlorous Acid
Abstract
Neutrophils kill invading microbes and therefore represent the first line of defense of the innate immune response. Activated neutrophils assemble NADPH oxidase to convert substantial amounts of molecular oxygen into superoxide, which, after dismutation into peroxide, serves as the substrate for the generation of the potent antimicrobial hypochlorous acid (HOCl) in the phagosomal space. In this minireview, we explore the most recent insights into physiological consequences of HOCl stress. Not surprisingly, Gram-negative bacteria have evolved diverse posttranslational defense mechanisms to protect their proteins, the main targets of HOCl, from HOCl-mediated damage. We discuss the idea that oxidation of conserved cysteine residues and partial unfolding of its structure convert the heat shock protein Hsp33 into a highly active chaperone holdase that binds unfolded proteins and prevents their aggregation. We examine two novel members of the Escherichia coli chaperone holdase family, RidA and CnoX, whose thiol-independent activation mechanism differs from that of Hsp33 and requires N-chlorination of positively charged amino acids during HOCl exposure. Furthermore, we summarize the latest findings with respect to another bacterial defense strategy employed in response to HOCl stress, which involves the accumulation of the universally conserved biopolymer inorganic polyphosphate. We then discuss sophisticated adaptive strategies that bacteria have developed to enhance their survival during HOCl stress. Understanding bacterial defense and survival strategies against one of the most powerful neutrophilic oxidants may provide novel insights into treatment options that potentially compromise the ability of pathogens to resist HOCl stress and therefore may increase the efficacy of the innate immune response.
Keywords: N-chlorination; biofilm; biofilm formation; chaperedoxin; disulfide bond formation; hypochlorous acid; molecular chaperone; motility; neutrophilic oxidants; oxidative burst; oxidative stress; polyphosphate; posttranslational modifications; protein aggregation.
Copyright © 2020 American Society for Microbiology.
Figures
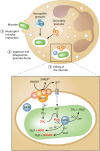
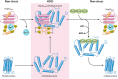
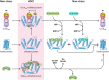
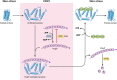
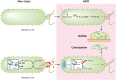
Similar articles
-
Stress-induced chaperones: a first line of defense against the powerful oxidant hypochlorous acid.F1000Res. 2019 Sep 23;8:F1000 Faculty Rev-1678. doi: 10.12688/f1000research.19517.1. eCollection 2019. F1000Res. 2019. PMID: 31583082 Free PMC article. Review.
-
The effects of neutrophil-generated hypochlorous acid and other hypohalous acids on host and pathogens.Cell Mol Life Sci. 2021 Jan;78(2):385-414. doi: 10.1007/s00018-020-03591-y. Epub 2020 Jul 13. Cell Mol Life Sci. 2021. PMID: 32661559 Free PMC article. Review.
-
Expression of RcrB confers resistance to hypochlorous acid in uropathogenic Escherichia coli.J Bacteriol. 2023 Oct 26;205(10):e0006423. doi: 10.1128/jb.00064-23. Epub 2023 Oct 4. J Bacteriol. 2023. PMID: 37791752 Free PMC article.
-
A Year at the Forefront of Bacterial Defense Systems Against Neutrophilic Oxidants.Biol Open. 2023 Apr 15;12(4):bio059809. doi: 10.1242/bio.059809. Epub 2023 Apr 27. Biol Open. 2023. PMID: 37102360 Free PMC article.
-
Bleach activates a redox-regulated chaperone by oxidative protein unfolding.Cell. 2008 Nov 14;135(4):691-701. doi: 10.1016/j.cell.2008.09.024. Cell. 2008. PMID: 19013278 Free PMC article.
Cited by
-
Abundant Monovalent Ions as Environmental Signposts for Pathogens during Host Colonization.Infect Immun. 2021 Mar 17;89(4):e00641-20. doi: 10.1128/IAI.00641-20. Print 2021 Mar 17. Infect Immun. 2021. PMID: 33526568 Free PMC article. Review.
-
Genome-Wide Screening of Oxidizing Agent Resistance Genes in Escherichia coli.Antioxidants (Basel). 2021 May 27;10(6):861. doi: 10.3390/antiox10060861. Antioxidants (Basel). 2021. PMID: 34072091 Free PMC article.
-
A universal polyphosphate kinase: PPK2c of Ralstonia eutropha accepts purine and pyrimidine nucleotides including uridine diphosphate.Appl Microbiol Biotechnol. 2020 Aug;104(15):6659-6667. doi: 10.1007/s00253-020-10706-9. Epub 2020 Jun 4. Appl Microbiol Biotechnol. 2020. PMID: 32500270 Free PMC article.
-
Escherichia coli RclA is a highly active hypothiocyanite reductase.Proc Natl Acad Sci U S A. 2022 Jul 26;119(30):e2119368119. doi: 10.1073/pnas.2119368119. Epub 2022 Jul 22. Proc Natl Acad Sci U S A. 2022. PMID: 35867824 Free PMC article.
-
Chlorine redox chemistry is widespread in microbiology.ISME J. 2023 Jan;17(1):70-83. doi: 10.1038/s41396-022-01317-5. Epub 2022 Oct 6. ISME J. 2023. PMID: 36202926 Free PMC article.
References
Publication types
MeSH terms
Substances
LinkOut - more resources
Full Text Sources
Medical
Miscellaneous