The Role of Carcinogenesis-Related Biomarkers in the Wnt Pathway and Their Effects on Epithelial-Mesenchymal Transition (EMT) in Oral Squamous Cell Carcinoma
- PMID: 32121061
- PMCID: PMC7139589
- DOI: 10.3390/cancers12030555
The Role of Carcinogenesis-Related Biomarkers in the Wnt Pathway and Their Effects on Epithelial-Mesenchymal Transition (EMT) in Oral Squamous Cell Carcinoma
Abstract
As oral squamous cell carcinoma (OSCC) can develop from potentially malignant disorders (PMDs), it is critical to develop methods for early detection to improve the prognosis of patients. Epithelial-mesenchymal transition (EMT) plays an important role during tumor progression and metastasis. The Wnt signaling pathway is an intercellular pathway in animals that also plays a fundamental role in cell proliferation and regeneration, and in the function of many cell or tissue types. Specific components of master regulators such as epithelial cadherin (E-cadherin), Vimentin, adenomatous polyposis coli (APC), Snail, and neural cadherin (N-cadherin), which are known to control the EMT process, have also been implicated in the Wnt cascade. Here, we review recent findings on the Wnt signaling pathway and the expression mechanism. These regulators are known to play roles in EMT and tumor progression, especially in OSCC. Characterizing the mechanisms through which both EMT and the Wnt pathway play a role in these cellular pathways could increase our understanding of the tumor genesis process and may allow for the development of improved therapeutics for OSCC.
Keywords: EMT; OSCC; PMD; WNT; biomarker.
Conflict of interest statement
The authors declare no conflicts of interest.
Figures
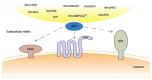
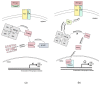
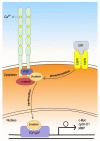
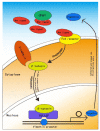
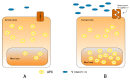
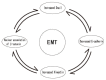
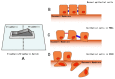
Similar articles
-
Epithelial to mesenchymal transition (EMT) biomarkers--E-cadherin, beta-catenin, APC and Vimentin--in oral squamous cell carcinogenesis and transformation.Oral Oncol. 2012 Oct;48(10):997-1006. doi: 10.1016/j.oraloncology.2012.05.011. Epub 2012 Jun 14. Oral Oncol. 2012. PMID: 22704062
-
Bovine lactoferrin reverses programming of epithelial-to-mesenchymal transition to mesenchymal-to-epithelial transition in oral squamous cell carcinoma.Biochem Biophys Res Commun. 2018 Dec 9;507(1-4):142-147. doi: 10.1016/j.bbrc.2018.10.193. Epub 2018 Nov 8. Biochem Biophys Res Commun. 2018. PMID: 30415774
-
HOXC10 promotes migration and invasion via the WNT-EMT signaling pathway in oral squamous cell carcinoma.J Cancer. 2019 Jul 25;10(19):4540-4551. doi: 10.7150/jca.30645. eCollection 2019. J Cancer. 2019. PMID: 31528218 Free PMC article.
-
Signaling pathways promoting epithelial mesenchymal transition in oral submucous fibrosis and oral squamous cell carcinoma.Jpn Dent Sci Rev. 2020 Nov;56(1):97-108. doi: 10.1016/j.jdsr.2020.07.002. Epub 2020 Aug 22. Jpn Dent Sci Rev. 2020. PMID: 32874377 Free PMC article. Review.
-
Epithelial-mesenchymal transition in ovarian cancer progression: a crucial role for the endothelin axis.Cells Tissues Organs. 2007;185(1-3):85-94. doi: 10.1159/000101307. Cells Tissues Organs. 2007. PMID: 17587812 Review.
Cited by
-
Epidemiology, Diagnostics, and Therapy of Oral Cancer-Update Review.Cancers (Basel). 2024 Sep 14;16(18):3156. doi: 10.3390/cancers16183156. Cancers (Basel). 2024. PMID: 39335128 Free PMC article. Review.
-
Curcuminoids as Modulators of EMT in Invasive Cancers: A Review of Molecular Targets With the Contribution of Malignant Mesothelioma Studies.Front Pharmacol. 2022 Jul 8;13:934534. doi: 10.3389/fphar.2022.934534. eCollection 2022. Front Pharmacol. 2022. PMID: 35873564 Free PMC article. Review.
-
Downregulation of THRSP Promotes Hepatocellular Carcinoma Progression by Triggering ZEB1 Transcription in an ERK-dependent Manner.J Cancer. 2021 May 19;12(14):4247-4256. doi: 10.7150/jca.51657. eCollection 2021. J Cancer. 2021. PMID: 34093825 Free PMC article.
-
Pseudopodium enriched atypical kinase 1(PEAK1) promotes invasion and of melanoma cells by activating JAK/STAT3 signals.Bioengineered. 2021 Dec;12(1):5045-5055. doi: 10.1080/21655979.2021.1961661. Bioengineered. 2021. PMID: 34365903 Free PMC article.
-
Systematic establishment and verification of an epithelial-mesenchymal transition gene signature for predicting prognosis of oral squamous cell carcinoma.Front Genet. 2023 Aug 10;14:1113137. doi: 10.3389/fgene.2023.1113137. eCollection 2023. Front Genet. 2023. PMID: 37636263 Free PMC article.
References
-
- Chaw S.Y., Abdul Majeed A., Dalley A.J., Chan A., Stein S., Farah C.S. Epithelial to mesenchymal transition (EMT) biomarkers—E-cadherin, beta-catenin, APC and Vimentin—In oral squamous cell carcinogenesis and transformation. Oral Oncol. 2012;48:997–1006. doi: 10.1016/j.oraloncology.2012.05.011. - DOI - PubMed
-
- Siu A., Lee C., Dang D., Lee C., Ramos D.M. Stem cell markers as predictors of oral cancer invasion. Anticancer Res. 2012;32:1163–1166. - PubMed
Publication types
LinkOut - more resources
Full Text Sources
Research Materials