Organ-on-a-chip: recent breakthroughs and future prospects
- PMID: 32050989
- PMCID: PMC7017614
- DOI: 10.1186/s12938-020-0752-0
Organ-on-a-chip: recent breakthroughs and future prospects
Abstract
The organ-on-a-chip (OOAC) is in the list of top 10 emerging technologies and refers to a physiological organ biomimetic system built on a microfluidic chip. Through a combination of cell biology, engineering, and biomaterial technology, the microenvironment of the chip simulates that of the organ in terms of tissue interfaces and mechanical stimulation. This reflects the structural and functional characteristics of human tissue and can predict response to an array of stimuli including drug responses and environmental effects. OOAC has broad applications in precision medicine and biological defense strategies. Here, we introduce the concepts of OOAC and review its application to the construction of physiological models, drug development, and toxicology from the perspective of different organs. We further discuss existing challenges and provide future perspectives for its application.
Keywords: Human organs; Microfluidic chip; Organ-on-a-chip; Physiological model; Stem cell.
Conflict of interest statement
The authors declare that they have no competing interests.
Figures
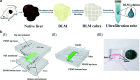
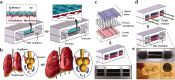
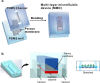
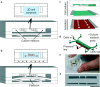
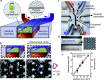
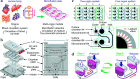
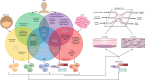
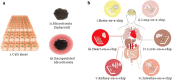
Similar articles
-
Breakthroughs and Applications of Organ-on-a-Chip Technology.Cells. 2022 Jun 2;11(11):1828. doi: 10.3390/cells11111828. Cells. 2022. PMID: 35681523 Free PMC article. Review.
-
Modelling Human Physiology on-Chip: Historical Perspectives and Future Directions.Micromachines (Basel). 2021 Oct 15;12(10):1250. doi: 10.3390/mi12101250. Micromachines (Basel). 2021. PMID: 34683301 Free PMC article. Review.
-
Microfluidic Organ-on-a-Chip System for Disease Modeling and Drug Development.Biosensors (Basel). 2022 May 27;12(6):370. doi: 10.3390/bios12060370. Biosensors (Basel). 2022. PMID: 35735518 Free PMC article. Review.
-
Organs-on-a-chip: a union of tissue engineering and microfabrication.Trends Biotechnol. 2023 Mar;41(3):410-424. doi: 10.1016/j.tibtech.2022.12.018. Epub 2023 Jan 31. Trends Biotechnol. 2023. PMID: 36725464 Free PMC article. Review.
-
Reconstitution of human tissue barrier function for precision and personalized medicine.Lab Chip. 2024 Jul 10;24(14):3347-3366. doi: 10.1039/d4lc00104d. Lab Chip. 2024. PMID: 38895863 Review.
Cited by
-
High-throughput Bronchus-on-a-Chip system for modeling the human bronchus.Sci Rep. 2024 Nov 1;14(1):26248. doi: 10.1038/s41598-024-77665-3. Sci Rep. 2024. PMID: 39482373 Free PMC article.
-
Overview of Biofluids and Flow Sensing Techniques Applied in Clinical Practice.Sensors (Basel). 2022 Sep 9;22(18):6836. doi: 10.3390/s22186836. Sensors (Basel). 2022. PMID: 36146183 Free PMC article. Review.
-
The convergence of high-tech emerging technologies into the next stage of organ-on-a-chips.Biomater Biosyst. 2021 Feb 20;1:100012. doi: 10.1016/j.bbiosy.2021.100012. eCollection 2021 Mar. Biomater Biosyst. 2021. PMID: 36825163 Free PMC article.
-
Direct Cell Reprogramming and Phenotypic Conversion: An Analysis of Experimental Attempts to Transform Astrocytes into Neurons in Adult Animals.Cells. 2023 Feb 14;12(4):618. doi: 10.3390/cells12040618. Cells. 2023. PMID: 36831283 Free PMC article. Review.
-
Modelling acute myeloid leukemia (AML): What's new? A transition from the classical to the modern.Drug Deliv Transl Res. 2023 Aug;13(8):2110-2141. doi: 10.1007/s13346-022-01189-4. Epub 2022 Aug 5. Drug Deliv Transl Res. 2023. PMID: 35930221 Free PMC article. Review.
References
Publication types
MeSH terms
Grants and funding
LinkOut - more resources
Full Text Sources