The Cryo-EM structure of pannexin 1 reveals unique motifs for ion selection and inhibition
- PMID: 32048993
- PMCID: PMC7108861
- DOI: 10.7554/eLife.54670
The Cryo-EM structure of pannexin 1 reveals unique motifs for ion selection and inhibition
Abstract
Pannexins are large-pore forming channels responsible for ATP release under a variety of physiological and pathological conditions. Although predicted to share similar membrane topology with other large-pore forming proteins such as connexins, innexins, and LRRC8, pannexins have minimal sequence similarity to these protein families. Here, we present the cryo-EM structure of a frog pannexin 1 (Panx1) channel at 3.0 Å. We find that Panx1 protomers harbor four transmembrane helices similar in arrangement to other large-pore forming proteins but assemble as a heptameric channel with a unique constriction formed by Trp74 in the first extracellular loop. Mutating Trp74 or the nearby Arg75 disrupt ion selectivity, whereas altering residues in the hydrophobic groove formed by the two extracellular loops abrogates channel inhibition by carbenoxolone. Our structural and functional study establishes the extracellular loops as important structural motifs for ion selectivity and channel inhibition in Panx1.
Keywords: ATP release; carbenoxolone; extracellular loop; heptameric channel; human; ion selectivity; molecular biophysics; neuroscience; pannexin; structural biology; xenopus.
© 2020, Michalski et al.
Conflict of interest statement
KM, JS, EH, JK, HF, TK No competing interests declared
Figures
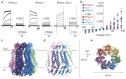
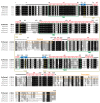
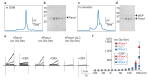
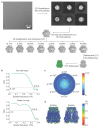
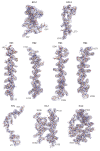
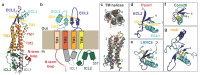
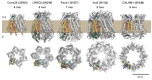
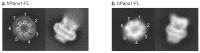
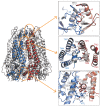
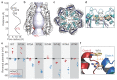
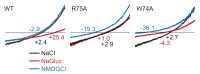
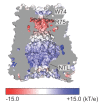
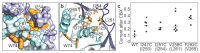
Comment in
-
Taking a close look at a large-pore channel.Elife. 2020 Mar 31;9:e56114. doi: 10.7554/eLife.56114. Elife. 2020. PMID: 32228857 Free PMC article.
Similar articles
-
Cryo-EM structures of the ATP release channel pannexin 1.Nat Struct Mol Biol. 2020 Apr;27(4):373-381. doi: 10.1038/s41594-020-0401-0. Epub 2020 Mar 30. Nat Struct Mol Biol. 2020. PMID: 32231289
-
Human Pannexin 1 channel: Insight in structure-function mechanism and its potential physiological roles.Mol Cell Biochem. 2021 Mar;476(3):1529-1540. doi: 10.1007/s11010-020-04002-3. Epub 2021 Jan 4. Mol Cell Biochem. 2021. PMID: 33394272 Review.
-
Single cysteines in the extracellular and transmembrane regions modulate pannexin 1 channel function.J Membr Biol. 2011 Nov;244(1):21-33. doi: 10.1007/s00232-011-9393-3. Epub 2011 Sep 21. J Membr Biol. 2011. PMID: 21938521
-
Emerging issues of connexin channels: biophysics fills the gap.Q Rev Biophys. 2001 Aug;34(3):325-472. doi: 10.1017/s0033583501003705. Q Rev Biophys. 2001. PMID: 11838236 Review.
-
SCAM analysis of Panx1 suggests a peculiar pore structure.J Gen Physiol. 2010 Nov;136(5):515-27. doi: 10.1085/jgp.201010440. Epub 2010 Oct 11. J Gen Physiol. 2010. PMID: 20937692 Free PMC article.
Cited by
-
Single-cell dynamics of pannexin-1-facilitated programmed ATP loss during apoptosis.Elife. 2020 Oct 14;9:e61960. doi: 10.7554/eLife.61960. Elife. 2020. PMID: 33052098 Free PMC article.
-
Pannexin-1 Contributes to the Apoptosis of Spinal Neurocytes in Spinal Cord Injury.Front Physiol. 2021 Apr 27;12:656647. doi: 10.3389/fphys.2021.656647. eCollection 2021. Front Physiol. 2021. PMID: 33986693 Free PMC article.
-
Pannexin-1 channel opening is critical for COVID-19 pathogenesis.iScience. 2021 Dec 17;24(12):103478. doi: 10.1016/j.isci.2021.103478. Epub 2021 Nov 19. iScience. 2021. PMID: 34841222 Free PMC article.
-
The application of Poisson distribution statistics in ion channel reconstitution to determine oligomeric architecture.Methods Enzymol. 2021;652:321-340. doi: 10.1016/bs.mie.2021.02.018. Epub 2021 Mar 27. Methods Enzymol. 2021. PMID: 34059289 Free PMC article.
-
Endothelial Pannexin 1 Channels Control Inflammation by Regulating Intracellular Calcium.J Immunol. 2020 Jun 1;204(11):2995-3007. doi: 10.4049/jimmunol.1901089. Epub 2020 Apr 20. J Immunol. 2020. PMID: 32312847 Free PMC article.
References
-
- Adams PD, Afonine PV, Bunkóczi G, Chen VB, Davis IW, Echols N, Headd JJ, Hung LW, Kapral GJ, Grosse-Kunstleve RW, McCoy AJ, Moriarty NW, Oeffner R, Read RJ, Richardson DC, Richardson JS, Terwilliger TC, Zwart PH. PHENIX: a comprehensive Python-based system for macromolecular structure solution. Acta Crystallographica Section D Biological Crystallography. 2010;66:213–221. doi: 10.1107/S0907444909052925. - DOI - PMC - PubMed
-
- Billaud M, Lohman AW, Straub AC, Looft-Wilson R, Johnstone SR, Araj CA, Best AK, Chekeni FB, Ravichandran KS, Penuela S, Laird DW, Isakson BE. Pannexin1 regulates α1-adrenergic receptor- mediated vasoconstriction. Circulation Research. 2011;109:80–85. doi: 10.1161/CIRCRESAHA.110.237594. - DOI - PMC - PubMed
-
- Billaud M, Chiu YH, Lohman AW, Parpaite T, Butcher JT, Mutchler SM, DeLalio LJ, Artamonov MV, Sandilos JK, Best AK, Somlyo AV, Thompson RJ, Le TH, Ravichandran KS, Bayliss DA, Isakson BE. A molecular signature in the pannexin1 intracellular loop confers channel activation by the α1 adrenoreceptor in smooth muscle cells. Science Signaling. 2015;8:ra17. doi: 10.1126/scisignal.2005824. - DOI - PMC - PubMed
Publication types
MeSH terms
Substances
Associated data
- Actions
Grants and funding
LinkOut - more resources
Full Text Sources
Other Literature Sources