A cyclin-dependent kinase, CDK11/p58, represses cap-dependent translation during mitosis
- PMID: 32030451
- PMCID: PMC7599166
- DOI: 10.1007/s00018-019-03436-3
A cyclin-dependent kinase, CDK11/p58, represses cap-dependent translation during mitosis
Abstract
During mitosis, translation of most mRNAs is strongly repressed; none of the several explanatory hypotheses suggested can fully explain the molecular basis of this phenomenon. Here we report that cyclin-dependent CDK11/p58-a serine/threonine kinase abundantly expressed during M phase-represses overall translation by phosphorylating a subunit (eIF3F) of the translation factor eIF3 complex that is essential for translation initiation of most mRNAs. Ectopic expression of CDK11/p58 strongly repressed cap-dependent translation, and knockdown of CDK11/p58 nullified the translational repression during M phase. We identified the phosphorylation sites in eIF3F responsible for M phase-specific translational repression by CDK11/p58. Alanine substitutions of CDK11/p58 target sites in eIF3F nullified its effects on cell cycle-dependent translational regulation. The mechanism of translational regulation by the M phase-specific kinase, CDK11/p58, has deep evolutionary roots considering the conservation of CDK11 and its target sites on eIF3F from C. elegans to humans.
Keywords: CDK11/p58; Translation initiation; Translational repression in M phase; eIF3F.
Conflict of interest statement
The authors declare no competing interests.
Figures
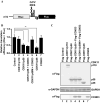
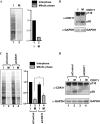
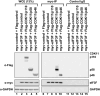
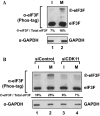





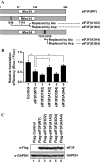
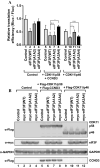
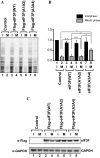
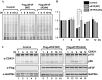
Similar articles
-
CDK11(p58) kinase activity is required to protect sister chromatid cohesion at centromeres in mitosis.Chromosome Res. 2014 Sep;22(3):267-76. doi: 10.1007/s10577-013-9400-x. Epub 2014 Jan 17. Chromosome Res. 2014. PMID: 24436071
-
CDK11(p58) is required for the maintenance of sister chromatid cohesion.J Cell Sci. 2007 Jul 15;120(Pt 14):2424-34. doi: 10.1242/jcs.007963. J Cell Sci. 2007. PMID: 17606997
-
Phosphorylation of the eukaryotic initiation factor 3f by cyclin-dependent kinase 11 during apoptosis.FEBS Lett. 2009 Mar 18;583(6):971-7. doi: 10.1016/j.febslet.2009.02.028. Epub 2009 Feb 24. FEBS Lett. 2009. PMID: 19245811 Free PMC article.
-
The Emerging Picture of CDK11: Genetic, Functional and Medicinal Aspects.Curr Med Chem. 2018;25(8):880-888. doi: 10.2174/0929867324666170815102036. Curr Med Chem. 2018. PMID: 28814241 Review.
-
The emerging roles and therapeutic potential of cyclin-dependent kinase 11 (CDK11) in human cancer.Oncotarget. 2016 Jun 28;7(26):40846-40859. doi: 10.18632/oncotarget.8519. Oncotarget. 2016. PMID: 27049727 Free PMC article. Review.
Cited by
-
Cyclin B/CDK1 and Cyclin A/CDK2 phosphorylate DENR to promote mitotic protein translation and faithful cell division.Nat Commun. 2022 Feb 3;13(1):668. doi: 10.1038/s41467-022-28265-0. Nat Commun. 2022. PMID: 35115540 Free PMC article.
-
Synergistic induction of mitotic pyroptosis and tumor remission by inhibiting proteasome and WEE family kinases.Signal Transduct Target Ther. 2024 Jul 12;9(1):181. doi: 10.1038/s41392-024-01896-z. Signal Transduct Target Ther. 2024. PMID: 38992067 Free PMC article.
-
The HIPK2/CDC14B-MeCP2 axis enhances the spindle assembly checkpoint block by promoting cyclin B translation.Sci Adv. 2023 Jan 20;9(3):eadd6982. doi: 10.1126/sciadv.add6982. Epub 2023 Jan 20. Sci Adv. 2023. PMID: 36662865 Free PMC article.
-
Cyclin-dependent kinases: Masters of the eukaryotic universe.Wiley Interdiscip Rev RNA. 2023 Sep 17;15(1):e1816. doi: 10.1002/wrna.1816. Online ahead of print. Wiley Interdiscip Rev RNA. 2023. PMID: 37718413 Free PMC article. Review.
-
Mitochondrial ribosomal small subunit (MRPS) MRPS23 protein-protein interaction reveals phosphorylation by CDK11-p58 affecting cell proliferation and knockdown of MRPS23 sensitizes breast cancer cells to CDK1 inhibitors.Mol Biol Rep. 2022 Oct;49(10):9521-9534. doi: 10.1007/s11033-022-07842-y. Epub 2022 Aug 13. Mol Biol Rep. 2022. PMID: 35962848
References
-
- Ron D, Harding HP (2007) eIF2α Phosphorylation in cellular stress responses and disease. In: Sonenberg N, Hershey JW, Mathews MB (eds) Translational control in biology and medicine. Cold Spring Harbor Laboratory Press, Cold Spring Harbor, pp 349–372. 10.1101/087969767.48.345 - DOI
MeSH terms
Substances
Grants and funding
LinkOut - more resources
Full Text Sources
Molecular Biology Databases
Research Materials
Miscellaneous