Methionine sulfoxide reductase B from Corynebacterium diphtheriae catalyzes sulfoxide reduction via an intramolecular disulfide cascade
- PMID: 31992594
- PMCID: PMC7076214
- DOI: 10.1074/jbc.RA119.012438
Methionine sulfoxide reductase B from Corynebacterium diphtheriae catalyzes sulfoxide reduction via an intramolecular disulfide cascade
Abstract
Corynebacterium diphtheriae is a human pathogen that causes diphtheria. In response to immune system-induced oxidative stress, C. diphtheriae expresses antioxidant enzymes, among which are methionine sulfoxide reductase (Msr) enzymes, which are critical for bacterial survival in the face of oxidative stress. Although some aspects of the catalytic mechanism of the Msr enzymes have been reported, several details still await full elucidation. Here, we solved the solution structure of C. diphtheriae MsrB (Cd-MsrB) and unraveled its catalytic and oxidation-protection mechanisms. Cd-MsrB catalyzes methionine sulfoxide reduction involving three redox-active cysteines. Using NMR heteronuclear single-quantum coherence spectra, kinetics, biochemical assays, and MS analyses, we show that the conserved nucleophilic residue Cys-122 is S-sulfenylated after substrate reduction, which is then resolved by a conserved cysteine, Cys-66, or by the nonconserved residue Cys-127. We noted that the overall structural changes during the disulfide cascade expose the Cys-122-Cys-66 disulfide to recycling through thioredoxin. In the presence of hydrogen peroxide, Cd-MsrB formed reversible intra- and intermolecular disulfides without losing its Cys-coordinated Zn2+, and only the nonconserved Cys-127 reacted with the low-molecular-weight (LMW) thiol mycothiol, protecting it from overoxidation. In summary, our structure-function analyses reveal critical details of the Cd-MsrB catalytic mechanism, including a major structural rearrangement that primes the Cys-122-Cys-66 disulfide for thioredoxin reduction and a reversible protection against excessive oxidation of the catalytic cysteines in Cd-MsrB through intra- and intermolecular disulfide formation and S-mycothiolation.
Keywords: biochemistry; enzyme mechanism; enzyme structure; hydrogen peroxide; kinetics; methionine sulfoxide; nuclear magnetic resonance (NMR); redox regulation.
© 2020 Tossounian et al.
Conflict of interest statement
The authors declare that they have no conflicts of interest with the contents of this article
Figures
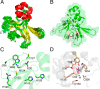
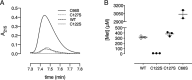
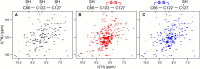
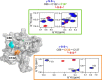
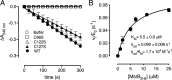
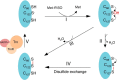
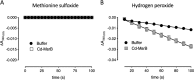
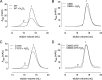
Similar articles
-
Corynebacterium diphtheriae methionine sulfoxide reductase a exploits a unique mycothiol redox relay mechanism.J Biol Chem. 2015 May 1;290(18):11365-75. doi: 10.1074/jbc.M114.632596. Epub 2015 Mar 9. J Biol Chem. 2015. PMID: 25752606 Free PMC article.
-
Functional comparison of methionine sulphoxide reductase A and B in Corynebacterium glutamicum.J Gen Appl Microbiol. 2017 Nov 17;63(5):280-286. doi: 10.2323/jgam.2017.01.005. Epub 2017 Sep 12. J Gen Appl Microbiol. 2017. PMID: 28904252
-
Reaction mechanism, evolutionary analysis, and role of zinc in Drosophila methionine-R-sulfoxide reductase.J Biol Chem. 2002 Oct 4;277(40):37527-35. doi: 10.1074/jbc.M203496200. Epub 2002 Jul 26. J Biol Chem. 2002. PMID: 12145281
-
Methionine sulfoxide reductase: chemistry, substrate binding, recycling process and oxidase activity.Bioorg Chem. 2014 Dec;57:222-230. doi: 10.1016/j.bioorg.2014.07.002. Epub 2014 Jul 24. Bioorg Chem. 2014. PMID: 25108804 Review.
-
Chemistry and Redox Biology of Mycothiol.Antioxid Redox Signal. 2018 Feb 20;28(6):487-504. doi: 10.1089/ars.2017.7074. Epub 2017 May 10. Antioxid Redox Signal. 2018. PMID: 28372502 Review.
Cited by
-
PKR activity modulation by phosphomimetic mutations of serine residues located three aminoacids upstream of double-stranded RNA binding motifs.Sci Rep. 2021 Apr 28;11(1):9188. doi: 10.1038/s41598-021-88610-z. Sci Rep. 2021. PMID: 33911136 Free PMC article.
-
Bacillus subtilis YtpP and Thioredoxin A Are New Players in the Coenzyme-A-Mediated Defense Mechanism against Cellular Stress.Antioxidants (Basel). 2023 Apr 15;12(4):938. doi: 10.3390/antiox12040938. Antioxidants (Basel). 2023. PMID: 37107313 Free PMC article.
-
A Year at the Forefront of Bacterial Defense Systems Against Neutrophilic Oxidants.Biol Open. 2023 Apr 15;12(4):bio059809. doi: 10.1242/bio.059809. Epub 2023 Apr 27. Biol Open. 2023. PMID: 37102360 Free PMC article.
-
Identification and Quantification of S-Sulfenylation Proteome of Mycobacterium tuberculosis under Oxidative Stress.Microbiol Spectr. 2023 Mar 21;11(2):e0338622. doi: 10.1128/spectrum.03386-22. Online ahead of print. Microbiol Spectr. 2023. PMID: 36943050 Free PMC article.
-
Profiling the Site of Protein CoAlation and Coenzyme A Stabilization Interactions.Antioxidants (Basel). 2022 Jul 14;11(7):1362. doi: 10.3390/antiox11071362. Antioxidants (Basel). 2022. PMID: 35883853 Free PMC article.
References
Publication types
MeSH terms
Substances
Associated data
- Actions
- Actions
- Actions
LinkOut - more resources
Full Text Sources