Structure of a rabies virus polymerase complex from electron cryo-microscopy
- PMID: 31953264
- PMCID: PMC6995008
- DOI: 10.1073/pnas.1918809117
Structure of a rabies virus polymerase complex from electron cryo-microscopy
Abstract
Nonsegmented negative-stranded (NNS) RNA viruses, among them the virus that causes rabies (RABV), include many deadly human pathogens. The large polymerase (L) proteins of NNS RNA viruses carry all of the enzymatic functions required for viral messenger RNA (mRNA) transcription and replication: RNA polymerization, mRNA capping, and cap methylation. We describe here a complete structure of RABV L bound with its phosphoprotein cofactor (P), determined by electron cryo-microscopy at 3.3 Å resolution. The complex closely resembles the vesicular stomatitis virus (VSV) L-P, the one other known full-length NNS-RNA L-protein structure, with key local differences (e.g., in L-P interactions). Like the VSV L-P structure, the RABV complex analyzed here represents a preinitiation conformation. Comparison with the likely elongation state, seen in two structures of pneumovirus L-P complexes, suggests differences between priming/initiation and elongation complexes. Analysis of internal cavities within RABV L suggests distinct template and product entry and exit pathways during transcription and replication.
Keywords: NNS RNA viruses; rabies lyssavirus; replication; transcription; vesicular stomatitis virus.
Copyright © 2020 the Author(s). Published by PNAS.
Conflict of interest statement
The authors declare no competing interest.
Figures
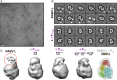
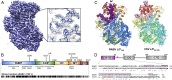
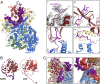
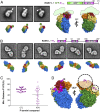
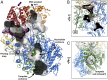
Comment in
-
Insight into the multifunctional RNA synthesis machine of rabies virus.Proc Natl Acad Sci U S A. 2020 Feb 25;117(8):3895-3897. doi: 10.1073/pnas.2000120117. Epub 2020 Jan 28. Proc Natl Acad Sci U S A. 2020. PMID: 31992635 Free PMC article. No abstract available.
Similar articles
-
Dimerization of Rabies Virus Phosphoprotein and Phosphorylation of Its Nucleoprotein Enhance Their Binding Affinity.Viruses. 2024 Nov 4;16(11):1735. doi: 10.3390/v16111735. Viruses. 2024. PMID: 39599850 Free PMC article.
-
The Structure of the Human Respiratory Syncytial Virus M2-1 Protein Bound to the Interaction Domain of the Phosphoprotein P Defines the Orientation of the Complex.mBio. 2018 Nov 13;9(6):e01554-18. doi: 10.1128/mBio.01554-18. mBio. 2018. PMID: 30425144 Free PMC article.
-
Cryo-EM structure of Nipah virus L-P polymerase complex.Nat Commun. 2024 Dec 3;15(1):10524. doi: 10.1038/s41467-024-54994-5. Nat Commun. 2024. PMID: 39627254 Free PMC article.
-
Depressing time: Waiting, melancholia, and the psychoanalytic practice of care.In: Kirtsoglou E, Simpson B, editors. The Time of Anthropology: Studies of Contemporary Chronopolitics. Abingdon: Routledge; 2020. Chapter 5. In: Kirtsoglou E, Simpson B, editors. The Time of Anthropology: Studies of Contemporary Chronopolitics. Abingdon: Routledge; 2020. Chapter 5. PMID: 36137063 Free Books & Documents. Review.
-
Antioxidants for female subfertility.Cochrane Database Syst Rev. 2017 Jul 28;7(7):CD007807. doi: 10.1002/14651858.CD007807.pub3. Cochrane Database Syst Rev. 2017. Update in: Cochrane Database Syst Rev. 2020 Aug 27;8:CD007807. doi: 10.1002/14651858.CD007807.pub4 PMID: 28752910 Free PMC article. Updated. Review.
Cited by
-
Negri bodies and other virus membrane-less replication compartments.Biochim Biophys Acta Mol Cell Res. 2020 Dec;1867(12):118831. doi: 10.1016/j.bbamcr.2020.118831. Epub 2020 Aug 21. Biochim Biophys Acta Mol Cell Res. 2020. PMID: 32835749 Free PMC article. Review.
-
GDP polyribonucleotidyltransferase domain of vesicular stomatitis virus polymerase regulates leader-promoter escape and polyadenylation-coupled termination during stop-start transcription.PLoS Pathog. 2022 Feb 2;18(2):e1010287. doi: 10.1371/journal.ppat.1010287. eCollection 2022 Feb. PLoS Pathog. 2022. PMID: 35108335 Free PMC article.
-
Structural basis of paramyxo- and pneumovirus polymerase inhibition by non-nucleoside small-molecule antivirals.Antimicrob Agents Chemother. 2024 Oct 8;68(10):e0080024. doi: 10.1128/aac.00800-24. Epub 2024 Aug 20. Antimicrob Agents Chemother. 2024. PMID: 39162479 Review.
-
Orally efficacious lead of the AVG inhibitor series targeting a dynamic interface in the respiratory syncytial virus polymerase.Sci Adv. 2022 Jun 24;8(25):eabo2236. doi: 10.1126/sciadv.abo2236. Epub 2022 Jun 24. Sci Adv. 2022. PMID: 35749502 Free PMC article.
-
Comparison of RNA synthesis initiation properties of non-segmented negative strand RNA virus polymerases.PLoS Pathog. 2021 Dec 16;17(12):e1010151. doi: 10.1371/journal.ppat.1010151. eCollection 2021 Dec. PLoS Pathog. 2021. PMID: 34914795 Free PMC article.
References
Publication types
MeSH terms
Substances
Associated data
- Actions
Grants and funding
LinkOut - more resources
Full Text Sources
Miscellaneous