Repeated Exposure to Multiple Concurrent Stresses Induce Circuit Specific Loss of Inputs to the Posterior Parietal Cortex
- PMID: 31949108
- PMCID: PMC7046453
- DOI: 10.1523/JNEUROSCI.1838-19.2020
Repeated Exposure to Multiple Concurrent Stresses Induce Circuit Specific Loss of Inputs to the Posterior Parietal Cortex
Abstract
Severe loss of excitatory synapses in key brain regions is thought to be one of the major mechanisms underlying stress-induced cognitive impairment. To date, however, the identity of the affected circuits remains elusive. Here we examined the effect of exposure to repeated multiple concurrent stressors (RMS) on the connectivity of the posterior parietal cortex (PPC) in adolescent male mice. We found that RMS led to layer-specific elimination of excitatory synapses with the most pronounced loss observed in deeper cortical layers. Quantitative analysis of cortical projections to the PPC revealed a significant loss of sensory and retrosplenial inputs to the PPC while contralateral and frontal projections were preserved. These results were confirmed by decreased synaptic strength from sensory, but not from contralateral, projections in stress-exposed animals. Functionally, RMS disrupted visuospatial working memory performance, implicating disrupted higher-order visual processing. These effects were not observed in mice subjected to restraint-only stress for an identical period of time. The PPC is considered to be a cortical hub for multisensory integration, working memory, and perceptual decision-making. Our data suggest that sensory information streams targeting the PPC may be impacted by recurring stress, likely contributing to stress-induced cognitive impairment.SIGNIFICANCE STATEMENT Repeated exposure to stress profoundly impairs cognitive functions like memory, attention, or decision-making. There is emerging evidence that stress not only impacts high-order regions of the brain, but may affect earlier stages of cognitive processing. Our work focuses on the posterior parietal cortex, a brain region supporting short-term memory, multisensory integration, and decision-making. We show evidence that repeated stress specifically damages sensory inputs to this region. This disruption of synaptic connectivity is linked to working memory impairment and is specific to repeated exposure to multiple stressors. Altogether, our data provide a potential alternative explanation to ailments previously attributed to downstream, cognitive brain structures.
Keywords: chronic stress; multimodal stress; posterior parietal cortex; retrograde tracing; synapse loss; visuospatial working memory.
Copyright © 2020 the authors.
Figures
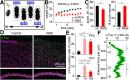
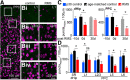
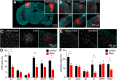
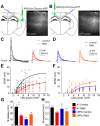
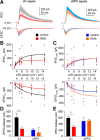
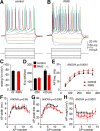
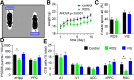
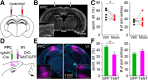
Similar articles
-
Repeated exposure to multiple concurrent stressors alters visual processing in the adult posterior parietal cortex.Neurobiol Stress. 2024 Jul 5;31:100660. doi: 10.1016/j.ynstr.2024.100660. eCollection 2024 Jul. Neurobiol Stress. 2024. PMID: 39100726 Free PMC article.
-
Working Memory and Decision-Making in a Frontoparietal Circuit Model.J Neurosci. 2017 Dec 13;37(50):12167-12186. doi: 10.1523/JNEUROSCI.0343-17.2017. Epub 2017 Nov 7. J Neurosci. 2017. PMID: 29114071 Free PMC article.
-
A Corticocortical Circuit Directly Links Retrosplenial Cortex to M2 in the Mouse.J Neurosci. 2016 Sep 7;36(36):9365-74. doi: 10.1523/JNEUROSCI.1099-16.2016. J Neurosci. 2016. PMID: 27605612 Free PMC article.
-
Visual perception is dependent on visuospatial working memory and thus on the posterior parietal cortex.Ann Phys Rehabil Med. 2017 Jun;60(3):141-147. doi: 10.1016/j.rehab.2016.01.002. Epub 2016 Feb 23. Ann Phys Rehabil Med. 2017. PMID: 26926263 Review.
-
The mouse posterior parietal cortex: Anatomy and functions.Neurosci Res. 2019 Mar;140:14-22. doi: 10.1016/j.neures.2018.10.008. Epub 2018 Nov 20. Neurosci Res. 2019. PMID: 30465783 Review.
Cited by
-
Unexpected Role of Physiological Estrogen in Acute Stress-Induced Memory Deficits.J Neurosci. 2021 Jan 27;41(4):648-662. doi: 10.1523/JNEUROSCI.2146-20.2020. Epub 2020 Dec 1. J Neurosci. 2021. PMID: 33262247 Free PMC article.
-
Sex-dependent long-term effects of prepubescent stress on the posterior parietal cortex.Neurobiol Stress. 2021 Jan 13;14:100295. doi: 10.1016/j.ynstr.2021.100295. eCollection 2021 May. Neurobiol Stress. 2021. PMID: 33521171 Free PMC article.
-
Effects of early life stress and subsequent re-exposure to stress on neuronal activity in the lateral habenula.Neuropsychopharmacology. 2023 Apr;48(5):745-753. doi: 10.1038/s41386-022-01493-0. Epub 2022 Nov 12. Neuropsychopharmacology. 2023. PMID: 36371544 Free PMC article.
-
Repeated exposure to multiple concurrent stressors alters visual processing in the adult posterior parietal cortex.Neurobiol Stress. 2024 Jul 5;31:100660. doi: 10.1016/j.ynstr.2024.100660. eCollection 2024 Jul. Neurobiol Stress. 2024. PMID: 39100726 Free PMC article.
-
Multiple Simultaneous Acute Stresses in Mice: Single or Repeated Induction.Bio Protoc. 2020 Aug 5;10(15):e3699. doi: 10.21769/BioProtoc.3699. eCollection 2020 Aug 5. Bio Protoc. 2020. PMID: 33659365 Free PMC article.
References
Publication types
MeSH terms
Substances
LinkOut - more resources
Full Text Sources
Other Literature Sources
Medical
Research Materials