Type II Toxin-Antitoxin Systems: Evolution and Revolutions
- PMID: 31932311
- PMCID: PMC7167474
- DOI: 10.1128/JB.00763-19
Type II Toxin-Antitoxin Systems: Evolution and Revolutions
Abstract
Type II toxin-antitoxin (TA) systems are small genetic elements composed of a toxic protein and its cognate antitoxin protein, the latter counteracting the toxicity of the former. While TA systems were initially discovered on plasmids, functioning as addiction modules through a phenomenon called postsegregational killing, they were later shown to be massively present in bacterial chromosomes, often in association with mobile genetic elements. Extensive research has been conducted in recent decades to better understand the physiological roles of these chromosomally encoded modules and to characterize the conditions leading to their activation. The diversity of their proposed roles, ranging from genomic stabilization and abortive phage infection to stress modulation and antibiotic persistence, in conjunction with the poor understanding of TA system regulation, resulted in the generation of simplistic models, often refuted by contradictory results. This review provides an epistemological and critical retrospective on TA modules and highlights fundamental questions concerning their roles and regulations that still remain unanswered.
Keywords: persistence; proteolysis; stress responses; toxin-antitoxin; transcriptional regulation.
Copyright © 2020 Fraikin et al.
Figures
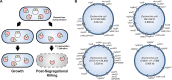
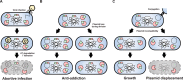
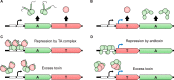
Similar articles
-
Genome-Wide Screening for Identification of Novel Toxin-Antitoxin Systems in Staphylococcus aureus.Appl Environ Microbiol. 2019 Oct 1;85(20):e00915-19. doi: 10.1128/AEM.00915-19. Print 2019 Oct 15. Appl Environ Microbiol. 2019. PMID: 31375497 Free PMC article.
-
PrrT/A, a Pseudomonas aeruginosa Bacterial Encoded Toxin-Antitoxin System Involved in Prophage Regulation and Biofilm Formation.Microbiol Spectr. 2022 Jun 29;10(3):e0118222. doi: 10.1128/spectrum.01182-22. Epub 2022 May 16. Microbiol Spectr. 2022. PMID: 35575497 Free PMC article.
-
Chromosomal toxin-antitoxin systems may act as antiaddiction modules.J Bacteriol. 2008 Jul;190(13):4603-9. doi: 10.1128/JB.00357-08. Epub 2008 Apr 25. J Bacteriol. 2008. PMID: 18441063 Free PMC article.
-
Toxin-Antitoxin Systems as Phage Defense Elements.Annu Rev Microbiol. 2022 Sep 8;76:21-43. doi: 10.1146/annurev-micro-020722-013730. Epub 2022 Apr 8. Annu Rev Microbiol. 2022. PMID: 35395167 Review.
-
Toxins, Targets, and Triggers: An Overview of Toxin-Antitoxin Biology.Mol Cell. 2018 Jun 7;70(5):768-784. doi: 10.1016/j.molcel.2018.01.003. Epub 2018 Feb 3. Mol Cell. 2018. PMID: 29398446 Review.
Cited by
-
Antibacterial T6SS effectors with a VRR-Nuc domain are structure-specific nucleases.Elife. 2022 Oct 13;11:e82437. doi: 10.7554/eLife.82437. Elife. 2022. PMID: 36226828 Free PMC article.
-
Targeting Type II Toxin-Antitoxin Systems as Antibacterial Strategies.Toxins (Basel). 2020 Sep 4;12(9):568. doi: 10.3390/toxins12090568. Toxins (Basel). 2020. PMID: 32899634 Free PMC article. Review.
-
tRNAfMet Inactivating Mycobacterium tuberculosis VapBC Toxin-Antitoxin Systems as Therapeutic Targets.Antimicrob Agents Chemother. 2022 May 17;66(5):e0189621. doi: 10.1128/aac.01896-21. Epub 2022 Apr 11. Antimicrob Agents Chemother. 2022. PMID: 35404073 Free PMC article.
-
Cellular Memory of HipA-Induced Growth Arrest: The Length of Cell Growth Arrest Becomes Shorter for Each Successive Induction.Microorganisms. 2021 Dec 15;9(12):2594. doi: 10.3390/microorganisms9122594. Microorganisms. 2021. PMID: 34946194 Free PMC article.
-
Modelling evolutionary pathways for commensalism and hypervirulence in Neisseria meningitidis.Microb Genom. 2021 Oct;7(10):000662. doi: 10.1099/mgen.0.000662. Microb Genom. 2021. PMID: 34704920 Free PMC article.
References
Publication types
MeSH terms
Substances
LinkOut - more resources
Full Text Sources
Molecular Biology Databases