Islet1 and Brn3 Expression Pattern Study in Human Retina and hiPSC-Derived Retinal Organoid
- PMID: 31885629
- PMCID: PMC6925930
- DOI: 10.1155/2019/8786396
Islet1 and Brn3 Expression Pattern Study in Human Retina and hiPSC-Derived Retinal Organoid
Abstract
This study was conducted to determine the dynamic Islet1 and Brn3 (POU4F) expression pattern in the human fetal retina and human-induced pluripotent stem cell- (hiPSC-) derived retinal organoid. Human fetal eyes from 8 to 27 fetal weeks (Fwks), human adult retina, hiPSC-derived retinal organoid from 7 to 31 differentiation weeks (Dwks), and rhesus adult retina were collected for cyrosectioning. Immunofluorescence analysis showed that Islet1 was expressed in retinal ganglion cells in the fetal retina, human adult retina, and retinal organoids. Unexpectedly, after Fwk 20, Brn3 expression gradually decreased in the fetal retina. In the midstage of development, Islet1 was detected in bipolar and developing horizontal cells. As the photoreceptor developed, the Islet1-positive cone precursors gradually became Islet1-negative/S-opsin-positive cones. This study highlights the distinguishing characteristics of Islet1 dynamic expression in human fetal retina development and proposes more concerns which should be taken regarding Brn3 as a cell-identifying marker in mature primate retina.
Copyright © 2019 Ziming Luo et al.
Conflict of interest statement
The authors declare no competing interests.
Figures
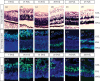
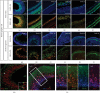
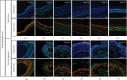
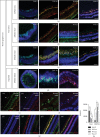
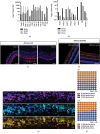
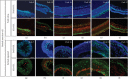
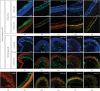
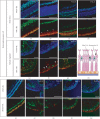
Similar articles
-
Expression of photoreceptor-associated molecules during human fetal eye development.Mol Vis. 2003 Aug 28;9:401-9. Mol Vis. 2003. PMID: 12949469
-
COCO enhances the efficiency of photoreceptor precursor differentiation in early human embryonic stem cell-derived retinal organoids.Stem Cell Res Ther. 2020 Aug 24;11(1):366. doi: 10.1186/s13287-020-01883-5. Stem Cell Res Ther. 2020. PMID: 32831148 Free PMC article.
-
Spatial and temporal expression of short, long/medium, or both opsins in human fetal cones.J Comp Neurol. 2000 Oct 2;425(4):545-59. J Comp Neurol. 2000. PMID: 10975879
-
Organoid technology for retinal repair.Dev Biol. 2018 Jan 15;433(2):132-143. doi: 10.1016/j.ydbio.2017.09.028. Epub 2017 Dec 25. Dev Biol. 2018. PMID: 29291970 Review.
-
Structural and Functional Characterization of Human Stem-Cell-Derived Retinal Organoids by Live Imaging.Invest Ophthalmol Vis Sci. 2017 Jul 1;58(9):3311-3318. doi: 10.1167/iovs.16-20796. Invest Ophthalmol Vis Sci. 2017. PMID: 28672397 Free PMC article. Review.
Cited by
-
Current trends and research topics regarding organoids: A bibliometric analysis of global research from 2000 to 2023.Heliyon. 2024 Jun 15;10(12):e32965. doi: 10.1016/j.heliyon.2024.e32965. eCollection 2024 Jun 30. Heliyon. 2024. PMID: 39022082 Free PMC article.
-
Cell replacement with stem cell-derived retinal ganglion cells from different protocols.Neural Regen Res. 2024 Apr;19(4):807-810. doi: 10.4103/1673-5374.381494. Neural Regen Res. 2024. PMID: 37843215 Free PMC article. Review.
-
Directly induced human retinal ganglion cells mimic fetal RGCs and are neuroprotective after transplantation in vivo.Stem Cell Reports. 2022 Dec 13;17(12):2690-2703. doi: 10.1016/j.stemcr.2022.10.011. Epub 2022 Nov 10. Stem Cell Reports. 2022. PMID: 36368332 Free PMC article.
-
Therapeutic strategies for glaucoma and optic neuropathies.Mol Aspects Med. 2023 Dec;94:101219. doi: 10.1016/j.mam.2023.101219. Epub 2023 Oct 13. Mol Aspects Med. 2023. PMID: 37839232 Free PMC article. Review.
-
Influence of hypoxia on retinal progenitor and ganglion cells in human induced pluripotent stem cell-derived retinal organoids.Int J Ophthalmol. 2023 Oct 18;16(10):1574-1581. doi: 10.18240/ijo.2023.10.03. eCollection 2023. Int J Ophthalmol. 2023. PMID: 37854379 Free PMC article.
References
LinkOut - more resources
Full Text Sources
Research Materials