Human microRNA-30 inhibits influenza virus infection by suppressing the expression of SOCS1, SOCS3, and NEDD4
- PMID: 31876380
- PMCID: PMC7162240
- DOI: 10.1111/cmi.13150
Human microRNA-30 inhibits influenza virus infection by suppressing the expression of SOCS1, SOCS3, and NEDD4
Abstract
Influenza A virus (IAV) has evolved multiple mechanisms to compromise type I interferon (IFN) responses. The antiviral function of IFN is mainly exerted by activating the JAK/STAT signalling and subsequently inducing IFN-stimulated gene (ISG) production. However, the mechanism by which IAV combat the type I IFN signalling pathway is not fully elucidated. In this study, we explored the roles of human microRNAs modulated by IAV infection in type I IFN responses. We demonstrated that microRNA-30 (miR-30) family members were downregulated by IAV infection. Our data showed that the forced expression of miR-30 family members inhibited IAV proliferation, while miR-30 family member inhibitors promoted IAV proliferation. Mechanistically, we found that miR-30 family members targeted and reduced SOCS1 and SOCS3 expression, and thus relieved their inhibiting effects on IFN/JAK/STAT signalling pathway. In addition, miR-30 family members inhibited the expression of NEDD4, a negative regulator of IFITM3, which is important for host defence against influenza viruses. Our findings suggest that IAV utilises a novel strategy to restrain host type I IFN-mediated antiviral immune responses by decreasing the expression of miR-30 family members, and add a new way to understand the mechanism of immune escape caused by influenza viruses.
Keywords: NEDD4; SOCS1; SOCS3; influenza virus; microRNA-30.
© 2019 John Wiley & Sons Ltd.
Conflict of interest statement
The authors declare no conflict of interest.
Figures
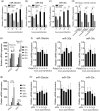
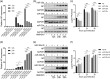
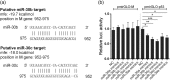
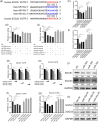
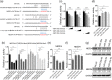
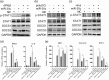
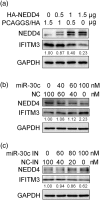
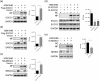
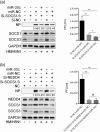
Similar articles
-
The Coronavirus Transmissible Gastroenteritis Virus Evades the Type I Interferon Response through IRE1α-Mediated Manipulation of the MicroRNA miR-30a-5p/SOCS1/3 Axis.J Virol. 2018 Oct 29;92(22):e00728-18. doi: 10.1128/JVI.00728-18. Print 2018 Nov 15. J Virol. 2018. PMID: 30185587 Free PMC article.
-
Downregulation of microRNA‑221 facilitates H1N1 influenza A virus replication through suppression of type‑IFN response by targeting the SOCS1/NF‑κB pathway.Mol Med Rep. 2021 Jul;24(1):497. doi: 10.3892/mmr.2021.12136. Epub 2021 May 6. Mol Med Rep. 2021. PMID: 33955508 Free PMC article.
-
Influenza a virus antagonizes type I and type II interferon responses via SOCS1-dependent ubiquitination and degradation of JAK1.Virol J. 2020 Jun 12;17(1):74. doi: 10.1186/s12985-020-01348-4. Virol J. 2020. PMID: 32532301 Free PMC article.
-
SOCS, Intrinsic Virulence Factors, and Treatment of COVID-19.Front Immunol. 2020 Oct 23;11:582102. doi: 10.3389/fimmu.2020.582102. eCollection 2020. Front Immunol. 2020. PMID: 33193390 Free PMC article. Review.
-
The Suppressor of Cytokine Signalling family of proteins and their potential impact on COVID-19 disease progression.Rev Med Virol. 2022 May;32(3):e2300. doi: 10.1002/rmv.2300. Epub 2021 Sep 21. Rev Med Virol. 2022. PMID: 34546610 Free PMC article. Review.
Cited by
-
SARS-CoV-2 produces a microRNA CoV2-miR-O8 in patients with COVID-19 infection.iScience. 2023 Dec 13;27(1):108719. doi: 10.1016/j.isci.2023.108719. eCollection 2024 Jan 19. iScience. 2023. PMID: 38226175 Free PMC article.
-
The Importance of miRNA Identification During Respiratory Viral Infections.J Cell Immunol. 2021;3(4):207-214. doi: 10.33696/immunology.3.101. J Cell Immunol. 2021. PMID: 34541575 Free PMC article.
-
MicroRNA-30a Modulates Type I Interferon Responses to Facilitate Coxsackievirus B3 Replication Via Targeting Tripartite Motif Protein 25.Front Immunol. 2021 Jan 14;11:603437. doi: 10.3389/fimmu.2020.603437. eCollection 2020. Front Immunol. 2021. PMID: 33519812 Free PMC article.
-
Role of microRNA in Endocrine Disruptor-Induced Immunomodulation of Metabolic Health.Metabolites. 2022 Oct 28;12(11):1034. doi: 10.3390/metabo12111034. Metabolites. 2022. PMID: 36355117 Free PMC article. Review.
-
Potential Molecular Mechanisms and Remdesivir Treatment for Acute Respiratory Syndrome Corona Virus 2 Infection/COVID 19 Through RNA Sequencing and Bioinformatics Analysis.Bioinform Biol Insights. 2021 Dec 23;15:11779322211067365. doi: 10.1177/11779322211067365. eCollection 2021. Bioinform Biol Insights. 2021. PMID: 34992355 Free PMC article.
References
Publication types
MeSH terms
Substances
LinkOut - more resources
Full Text Sources
Medical