Severe Fever with Thrombocytopenia Syndrome Virus NSs Interacts with TRIM21 To Activate the p62-Keap1-Nrf2 Pathway
- PMID: 31852783
- PMCID: PMC7158713
- DOI: 10.1128/JVI.01684-19
Severe Fever with Thrombocytopenia Syndrome Virus NSs Interacts with TRIM21 To Activate the p62-Keap1-Nrf2 Pathway
Abstract
Nuclear factor erythroid 2-related factor 2 (Nrf2) dissociates from its inhibitor, Keap1, upon stress signals and subsequently induces an antioxidant response that critically controls the viral life cycle and pathogenesis. Besides intracellular Fc receptor function, tripartite motif 21 (TRIM21) E3 ligase plays an essential role in the p62-Keap1-Nrf2 axis pathway for redox homeostasis. Specifically, TRIM21-mediated p62 ubiquitination abrogates p62 oligomerization and sequestration activity and negatively regulates the Keap1-Nrf2-mediated antioxidant response. A number of viruses target the Nrf2-mediated antioxidant response to generate an optimal environment for their life cycle. Here we report that a nonstructural protein (NSs) of severe fever with thrombocytopenia syndrome virus (SFTSV) interacts with and inhibits TRIM21 to activate the Nrf2 antioxidant signal pathway. Mass spectrometry identified TRIM21 to be a binding protein for NSs. NSs bound to the carboxyl-terminal SPRY subdomain of TRIM21, enhancing p62 stability and oligomerization. This facilitated p62-mediated Keap1 sequestration and ultimately increased Nrf2-mediated transcriptional activation of antioxidant genes, including those for heme oxygenase 1, NAD(P)H quinone oxidoreductase 1, and CD36. Mutational analysis found that the NSs-A46 mutant, which no longer interacted with TRIM21, was unable to increase Nrf2-mediated transcriptional activation. Functionally, the NS wild type (WT), but not the NSs-A46 mutant, increased the surface expression of the CD36 scavenger receptor, resulting in an increase in phagocytosis and lipid uptake. A combination of reverse genetics and assays with Ifnar-/- mouse models revealed that while the SFTSV-A46 mutant replicated similarly to wild-type SFTSV (SFTSV-WT), it showed weaker pathogenic activity than SFTSV-WT. These data suggest that the activation of the p62-Keap1-Nrf2 antioxidant response induced by the NSs-TRIM21 interaction contributes to the development of an optimal environment for the SFTSV life cycle and efficient pathogenesis.IMPORTANCE Tick-borne diseases have become a growing threat to public health. SFTSV, listed by the World Health Organization as a prioritized pathogen, is an emerging phlebovirus, and fatality rates among those infected with this virus are high. Infected Haemaphysalis longicornis ticks are the major source of human SFTSV infection. In particular, the recent spread of this tick to over 12 states in the United States has increased the potential for outbreaks of this disease beyond Far East Asia. Due to the lack of therapies and vaccines against SFTSV infection, there is a pressing need to understand SFTSV pathogenesis. As the Nrf2-mediated antioxidant response affects viral life cycles, a number of viruses deregulate Nrf2 pathways. Here we demonstrate that the SFTSV NSs inhibits the TRIM21 function to upregulate the p62-Keap1-Nrf2 antioxidant pathway for efficient viral pathogenesis. This study not only demonstrates the critical role of SFTSV NSs in viral pathogenesis but also suggests potential future therapeutic approaches to treat SFTSV-infected patients.
Keywords: Nrf2 pathway; SFTSV; TRIM21; nonstructural protein.
Copyright © 2020 American Society for Microbiology.
Figures
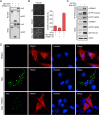
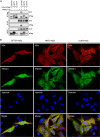
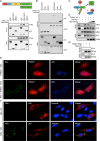
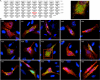
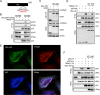
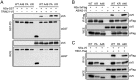
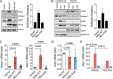
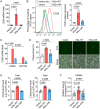
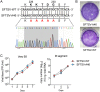
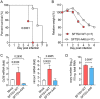
Similar articles
-
The Severe Fever with Thrombocytopenia Syndrome Virus NSs Protein Interacts with CDK1 To Induce G2 Cell Cycle Arrest and Positively Regulate Viral Replication.J Virol. 2020 Feb 28;94(6):e01575-19. doi: 10.1128/JVI.01575-19. Print 2020 Feb 28. J Virol. 2020. PMID: 31852787 Free PMC article.
-
The Ubiquitin E3 Ligase TRIM21 Promotes Hepatocarcinogenesis by Suppressing the p62-Keap1-Nrf2 Antioxidant Pathway.Cell Mol Gastroenterol Hepatol. 2021;11(5):1369-1385. doi: 10.1016/j.jcmgh.2021.01.007. Epub 2021 Jan 19. Cell Mol Gastroenterol Hepatol. 2021. PMID: 33482392 Free PMC article.
-
Severe fever with thrombocytopenia syndrome phlebovirus non-structural protein activates TPL2 signalling pathway for viral immunopathogenesis.Nat Microbiol. 2019 Mar;4(3):429-437. doi: 10.1038/s41564-018-0329-x. Epub 2019 Jan 7. Nat Microbiol. 2019. PMID: 30617349 Free PMC article.
-
Novel target for treating Alzheimer's Diseases: Crosstalk between the Nrf2 pathway and autophagy.Ageing Res Rev. 2021 Jan;65:101207. doi: 10.1016/j.arr.2020.101207. Epub 2020 Nov 1. Ageing Res Rev. 2021. PMID: 33144123 Review.
-
Sequestered SQSTM1/p62 crosstalk with Keap1/NRF2 axis in hPDLCs promotes oxidative stress injury induced by periodontitis.Free Radic Biol Med. 2022 Sep;190:62-74. doi: 10.1016/j.freeradbiomed.2022.08.001. Epub 2022 Aug 5. Free Radic Biol Med. 2022. PMID: 35940517 Review.
Cited by
-
Bunyavirus SFTSV exploits autophagic flux for viral assembly and egress.Autophagy. 2022 Jul;18(7):1599-1612. doi: 10.1080/15548627.2021.1994296. Epub 2021 Nov 6. Autophagy. 2022. PMID: 34747299 Free PMC article.
-
TRIM21 Promotes Oxidative Stress and Ferroptosis through the SQSTM1-NRF2-KEAP1 Axis to Increase the Titers of H5N1 Highly Pathogenic Avian Influenza Virus.Int J Mol Sci. 2024 Mar 14;25(6):3315. doi: 10.3390/ijms25063315. Int J Mol Sci. 2024. PMID: 38542289 Free PMC article.
-
A Look into Bunyavirales Genomes: Functions of Non-Structural (NS) Proteins.Viruses. 2021 Feb 18;13(2):314. doi: 10.3390/v13020314. Viruses. 2021. PMID: 33670641 Free PMC article. Review.
-
Severe fever with thrombocytopenia syndrome virus induces lactylation of m6A reader protein YTHDF1 to facilitate viral replication.EMBO Rep. 2024 Dec;25(12):5599-5619. doi: 10.1038/s44319-024-00310-7. Epub 2024 Nov 4. EMBO Rep. 2024. PMID: 39496835 Free PMC article.
-
TRIM Proteins in Host Defense and Viral Pathogenesis.Curr Clin Microbiol Rep. 2020;7(4):101-114. doi: 10.1007/s40588-020-00150-8. Epub 2020 Aug 8. Curr Clin Microbiol Rep. 2020. PMID: 32837832 Free PMC article. Review.
References
-
- Abudurexiti A, Adkins S, Alioto D, Alkhovsky SV, Avšič-Županc T, Ballinger MJ, Bente DA, Beer M, Bergeron É, Blair CD, Briese T, Buchmeier MJ, Burt FJ, Calisher CH, Cháng C, Charrel RN, Choi IR, Clegg JCS, de la Torre JC, de Lamballerie X, Dèng F, Di Serio F, Digiaro M, Drebot MA, Duàn X, Ebihara H, Elbeaino T, Ergünay K, Fulhorst CF, Garrison AR, Gāo GF, Gonzalez J-PJ, Groschup MH, Günther S, Haenni A-L, Hall RA, Hepojoki J, Hewson R, Hú Z, Hughes HR, Jonson MG, Junglen S, Klempa B, Klingström J, Kòu C, Laenen L, Lambert AJ, Langevin SA, Liu D, Lukashevich IS, et al. . 2019. Taxonomy of the order Bunyavirales: update 2019. Arch Virol 164:1949–1965. doi:10.1007/s00705-019-04253-6. - DOI - PMC - PubMed
-
- Yu XJ, Liang MF, Zhang SY, Liu Y, Li JD, Sun YL, Zhang L, Zhang QF, Popov VL, Li C, Qu J, Li Q, Zhang YP, Hai R, Wu W, Wang Q, Zhan FX, Wang XJ, Kan B, Wang SW, Wan KL, Jing HQ, Lu JX, Yin WW, Zhou H, Guan XH, Liu JF, Bi ZQ, Liu GH, Ren J, Wang H, Zhao Z, Song JD, He JR, Wan T, Zhang JS, Fu XP, Sun LN, Dong XP, Feng ZJ, Yang WZ, Hong T, Zhang Y, Walker DH, Wang Y, Li DX. 2011. Fever with thrombocytopenia associated with a novel bunyavirus in China. N Engl J Med 364:1523–1532. doi:10.1056/NEJMoa1010095. - DOI - PMC - PubMed
-
- Zhang YZ, He YW, Dai YA, Xiong Y, Zheng H, Zhou DJ, Li J, Sun Q, Luo XL, Cheng YL, Qin XC, Tian JH, Chen XP, Yu B, Jin D, Guo WP, Li W, Wang W, Peng JS, Zhang GB, Zhang S, Chen XM, Wang Y, Li MH, Li Z, Lu S, Ye C, de Jong MD, Xu J. 2012. Hemorrhagic fever caused by a novel bunyavirus in China: pathogenesis and correlates of fatal outcome. Clin Infect Dis 54:527–533. doi:10.1093/cid/cir804. - DOI - PubMed
-
- Xing X, Guan X, Liu L, Xu J, Li G, Zhan J, Liu G, Jiang X, Shen X, Jiang Y, Wu Y, Zhang H, Huang J, Ding F, Sha S, Liu M, Zhan F. 2017. A case-control study of risk sources for severe fever with thrombocytopenia syndrome in Hubei Province, China. Int J Infect Dis 55:86–91. doi:10.1016/j.ijid.2017.01.003. - DOI - PubMed
Publication types
MeSH terms
Substances
Grants and funding
LinkOut - more resources
Full Text Sources
Research Materials
Miscellaneous