Terminal Schwann cell and vacant site mediated synapse elimination at developing neuromuscular junctions
- PMID: 31819113
- PMCID: PMC6901572
- DOI: 10.1038/s41598-019-55017-w
Terminal Schwann cell and vacant site mediated synapse elimination at developing neuromuscular junctions
Abstract
Synapses undergo transition from polyinnervation by multiple axons to single innervation a few weeks after birth. Synaptic activity of axons and interaxonal competition are thought to drive this developmental synapse elimination and tested as key parameters in quantitative models for further understanding. Recent studies of muscle synapses (endplates) show that there are also terminal Schwann cells (tSCs), glial cells associated with motor neurons and their functions, and vacant sites (or vacancies) devoid of tSCs and axons proposing tSCs as key effectors of synapse elimination. However, there is no quantitative model that considers roles of tSCs including vacancies. Here we develop a stochastic model of tSC and vacancy mediated synapse elimination. It employs their areas on individual endplates quantified by electron microscopy-based analyses assuming that vacancies form randomly and are taken over by adjacent axons or tSCs. The model reliably reproduced synapse elimination whereas equal or random probability models, similar to classical interaxonal competition models, did not. Furthermore, the model showed that synapse elimination is accelerated by enhanced synaptic activity of one axon and also by increased areas of vacancies and tSCs suggesting that the areas are important structural correlates of the rate of synapse elimination.
Conflict of interest statement
The authors declare no competing interests.
Figures
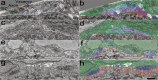
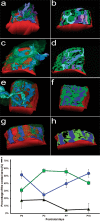
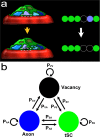
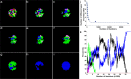
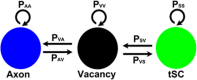
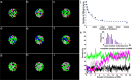
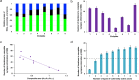
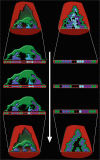
Similar articles
-
Neuregulin1 displayed on motor axons regulates terminal Schwann cell-mediated synapse elimination at developing neuromuscular junctions.Proc Natl Acad Sci U S A. 2016 Jan 26;113(4):E479-87. doi: 10.1073/pnas.1519156113. Epub 2016 Jan 11. Proc Natl Acad Sci U S A. 2016. PMID: 26755586 Free PMC article.
-
Testosterone regulates terminal Schwann cell number and junctional size during developmental synapse elimination.Dev Neurosci. 2001;23(6):441-51. doi: 10.1159/000048731. Dev Neurosci. 2001. PMID: 11872945
-
Developmental neuromuscular synapse elimination: Activity-dependence and potential downstream effector mechanisms.Neurosci Lett. 2020 Jan 23;718:134724. doi: 10.1016/j.neulet.2019.134724. Epub 2019 Dec 23. Neurosci Lett. 2020. PMID: 31877335 Free PMC article. Review.
-
Terminal Schwann cells participate in the competition underlying neuromuscular synapse elimination.J Neurosci. 2013 Nov 6;33(45):17724-36. doi: 10.1523/JNEUROSCI.3339-13.2013. J Neurosci. 2013. PMID: 24198364 Free PMC article.
-
Schwann cells participate in synapse elimination at the developing neuromuscular junction.Curr Opin Neurobiol. 2017 Dec;47:176-181. doi: 10.1016/j.conb.2017.10.010. Epub 2017 Nov 6. Curr Opin Neurobiol. 2017. PMID: 29121585 Free PMC article. Review.
Cited by
-
Neurexin and neuroligins jointly regulate synaptic degeneration at the Drosophila neuromuscular junction based on TEM studies.Front Cell Neurosci. 2023 Nov 1;17:1257347. doi: 10.3389/fncel.2023.1257347. eCollection 2023. Front Cell Neurosci. 2023. PMID: 38026694 Free PMC article.
-
Three-dimensional in vitro models of neuromuscular tissue.Neural Regen Res. 2022 Apr;17(4):759-766. doi: 10.4103/1673-5374.322447. Neural Regen Res. 2022. PMID: 34472462 Free PMC article. Review.
-
More Than Mortar: Glia as Architects of Nervous System Development and Disease.Front Cell Dev Biol. 2020 Dec 14;8:611269. doi: 10.3389/fcell.2020.611269. eCollection 2020. Front Cell Dev Biol. 2020. PMID: 33381506 Free PMC article. Review.
-
Neuromuscular Junction Development Differs Between Extraocular and Skeletal Muscles and Between Different Extraocular Muscles.Invest Ophthalmol Vis Sci. 2024 May 1;65(5):28. doi: 10.1167/iovs.65.5.28. Invest Ophthalmol Vis Sci. 2024. PMID: 38767908 Free PMC article.
-
Trigeminal Sensory Supply Is Essential for Motor Recovery after Facial Nerve Injury.Int J Mol Sci. 2022 Dec 1;23(23):15101. doi: 10.3390/ijms232315101. Int J Mol Sci. 2022. PMID: 36499425 Free PMC article. Review.
References
Publication types
MeSH terms
LinkOut - more resources
Full Text Sources