Nonequilibrium Biophysical Processes Influence the Large-Scale Architecture of the Cell Nucleus
- PMID: 31818465
- PMCID: PMC7202941
- DOI: 10.1016/j.bpj.2019.11.017
Nonequilibrium Biophysical Processes Influence the Large-Scale Architecture of the Cell Nucleus
Abstract
Model approaches to nuclear architecture have traditionally ignored the biophysical consequences of ATP-fueled active processes acting on chromatin. However, transcription-coupled activity is a source of stochastic forces that are substantially larger than the Brownian forces present at physiological temperatures. Here, we describe an approach to large-scale nuclear architecture in metazoans that incorporates cell-type-specific active processes. The model predicts the statistics of positional distributions, shapes, and overlaps of each chromosome. Simulations of the model reproduce common organizing principles underlying large-scale nuclear architecture across human cell nuclei in interphase. These include the differential positioning of euchromatin and heterochromatin, the territorial organization of chromosomes (including both gene-density-based and size-based chromosome radial positioning schemes), the nonrandom locations of chromosome territories, and the shape statistics of individual chromosomes. We propose that the biophysical consequences of the distribution of transcriptional activity across chromosomes should be central to any chromosome positioning code.
Copyright © 2019 Biophysical Society. Published by Elsevier Inc. All rights reserved.
Figures
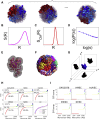
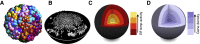
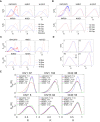
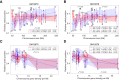
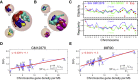
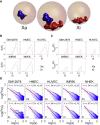
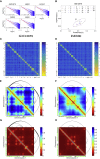
Similar articles
-
Chromatin as an active polymeric material.Emerg Top Life Sci. 2020 Sep 8;4(2):111-118. doi: 10.1042/ETLS20200010. Emerg Top Life Sci. 2020. PMID: 32830859
-
[Topology of chromosomes in somatic cells. Part 1].Postepy Hig Med Dosw (Online). 2006;60:331-42. Postepy Hig Med Dosw (Online). 2006. PMID: 16819432 Review. Polish.
-
Chromosome positioning from activity-based segregation.Nucleic Acids Res. 2014 Apr;42(7):4145-59. doi: 10.1093/nar/gkt1417. Epub 2014 Jan 22. Nucleic Acids Res. 2014. PMID: 24459132 Free PMC article.
-
Simulation of Different Three-Dimensional Models of Whole Interphase Nuclei Compared to Experiments - A Consistent Scale-Bridging Simulation Framework for Genome Organization.Results Probl Cell Differ. 2022;70:495-549. doi: 10.1007/978-3-031-06573-6_18. Results Probl Cell Differ. 2022. PMID: 36348120
-
Chromosome topology in mammalian interphase nuclei.Exp Cell Res. 1991 Feb;192(2):325-32. doi: 10.1016/0014-4827(91)90048-y. Exp Cell Res. 1991. PMID: 1988281 Review.
Cited by
-
Diffusiophoresis promotes phase separation and transport of biomolecular condensates.Nat Commun. 2024 Sep 3;15(1):7686. doi: 10.1038/s41467-024-51840-6. Nat Commun. 2024. PMID: 39227569 Free PMC article.
-
Diffusiophoresis promotes phase separation and transport of biomolecular condensates.bioRxiv [Preprint]. 2024 Feb 17:2023.07.03.547532. doi: 10.1101/2023.07.03.547532. bioRxiv. 2024. Update in: Nat Commun. 2024 Sep 3;15(1):7686. doi: 10.1038/s41467-024-51840-6 PMID: 37461689 Free PMC article. Updated. Preprint.
-
How enzymatic activity is involved in chromatin organization.Elife. 2022 Dec 6;11:e79901. doi: 10.7554/eLife.79901. Elife. 2022. PMID: 36472500 Free PMC article.
-
Phase Separation and Correlated Motions in Motorized Genome.J Phys Chem B. 2022 Aug 4;126(30):5619-5628. doi: 10.1021/acs.jpcb.2c03238. Epub 2022 Jul 20. J Phys Chem B. 2022. PMID: 35858189 Free PMC article.
-
Telomeres and Subtelomeres Dynamics in the Context of Early Chromosome Interactions During Meiosis and Their Implications in Plant Breeding.Front Plant Sci. 2021 Jun 4;12:672489. doi: 10.3389/fpls.2021.672489. eCollection 2021. Front Plant Sci. 2021. PMID: 34149773 Free PMC article. Review.
References
Publication types
MeSH terms
Substances
LinkOut - more resources
Full Text Sources