Porcine Alveolar Macrophages' Nitric Oxide Synthase-Mediated Generation of Nitric Oxide Exerts Important Defensive Effects against Glaesserella parasuis Infection
- PMID: 31766159
- PMCID: PMC6963498
- DOI: 10.3390/pathogens8040234
Porcine Alveolar Macrophages' Nitric Oxide Synthase-Mediated Generation of Nitric Oxide Exerts Important Defensive Effects against Glaesserella parasuis Infection
Abstract
Glaesserella parasuis is a habitual bacterium of pigs' upper respiratory tracts. Its infection initiates with the invasion and colonization of the lower respiratory tracts of pigs, and develops as the bacteria survive host pulmonary defenses and clearance by alveolar macrophages. Alveolar macrophage-derived nitric oxide (NO) is recognized as an important mediator that exerts antimicrobial activity as well as immunomodulatory effects. In this study, we investigated the effects and the signaling pathway of NO generation in porcine alveolar macrophages 3D4/21 during G. parasuis infection. We demonstrated a time and dose-dependent generation of NO in 3D4/21 cells by G. parasuis, and showed that NO production required bacterial viability and nitric oxide synthase 2 upregulation, which was largely contributed by G. parasuis-induced nuclear factor-κB signaling's activation. Moreover, the porcine alveolar macrophage-derived NO exhibited prominent bacteriostatic effects against G. parasuis and positive host immunomodulation effects by inducing the production of cytokines and chemokines during infection. G. parasuis in turn, selectively upregulated several nitrate reductase genes to better survive this NO stress, revealing a battle of wits during the bacteria-host interactions. To our knowledge, this is the first direct demonstration of NO production and its anti-infection effects in alveolar macrophages with G. parasuis infection.
Keywords: Glaesserella parasuis; NF-κB signaling; NOS2; nitric oxide; porcine alveolar macrophages.
Conflict of interest statement
The authors declare no conflict of interest.
Figures
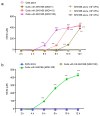
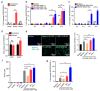
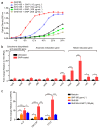
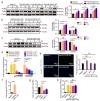
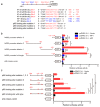
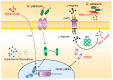
Similar articles
-
Glaesserella parasuis induces inflammatory response in 3D4/21 cells through activation of NLRP3 inflammasome signaling pathway via ROS.Vet Microbiol. 2021 May;256:109057. doi: 10.1016/j.vetmic.2021.109057. Epub 2021 Mar 26. Vet Microbiol. 2021. PMID: 33799227
-
HbpA from Glaesserella parasuis induces an inflammatory response in 3D4/21 cells by activating the MAPK and NF-κB signalling pathways and protects mice against G. parasuis when used as an immunogen.Vet Res. 2024 Jul 29;55(1):93. doi: 10.1186/s13567-024-01344-4. Vet Res. 2024. PMID: 39075605 Free PMC article.
-
Sialylated Lipooligosaccharide Contributes to Glaesserella parasuis Penetration of Porcine Respiratory Epithelial Barrier.ACS Infect Dis. 2021 Mar 12;7(3):661-671. doi: 10.1021/acsinfecdis.0c00850. Epub 2021 Mar 1. ACS Infect Dis. 2021. PMID: 33645216
-
Sialidase of Glaesserella parasuis Augments Inflammatory Response via Desialylation and Abrogation of Negative Regulation of Siglec-5.Infect Immun. 2021 Apr 16;89(5):e00696-20. doi: 10.1128/IAI.00696-20. Print 2021 Apr 16. Infect Immun. 2021. PMID: 33526563 Free PMC article.
-
Haemophilus parasuis: infection, immunity and enrofloxacin.Vet Res. 2015 Oct 28;46:128. doi: 10.1186/s13567-015-0263-3. Vet Res. 2015. PMID: 26511717 Free PMC article. Review.
References
-
- Costa-Hurtado M., Olvera A., Martinez-Moliner V., Galofre-Mila N., Martinez P., Dominguez J., Aragon V. Changes in macrophage phenotype after infection of pigs with Haemophilus parasuis strains with different levels of virulence. Infect. Immun. 2013;81:2327–2333. doi: 10.1128/IAI.00056-13. - DOI - PMC - PubMed
Grants and funding
LinkOut - more resources
Full Text Sources