Protein:Protein interactions in the cytoplasmic membrane apparently influencing sugar transport and phosphorylation activities of the e. coli phosphotransferase system
- PMID: 31751341
- PMCID: PMC6872149
- DOI: 10.1371/journal.pone.0219332
Protein:Protein interactions in the cytoplasmic membrane apparently influencing sugar transport and phosphorylation activities of the e. coli phosphotransferase system
Abstract
The multicomponent phosphoenolpyruvate (PEP)-dependent sugar-transporting phosphotransferase system (PTS) in Escherichia coli takes up sugar substrates from the medium and concomitantly phosphorylates them, releasing sugar phosphates into the cytoplasm. We have recently provided evidence that many of the integral membrane PTS permeases interact with the fructose PTS (FruA/FruB) [1]. However, the biochemical and physiological significance of this finding was not known. We have carried out molecular genetic/biochemical/physiological studies that show that interactions of the fructose PTS often enhance, but sometimes inhibit the activities of other PTS transporters many fold, depending on the target PTS system under study. Thus, the glucose (Glc), mannose (Man), mannitol (Mtl) and N-acetylglucosamine (NAG) permeases exhibit enhanced in vivo sugar transport and sometimes in vitro PEP-dependent sugar phosphorylation activities while the galactitol (Gat) and trehalose (Tre) systems show inhibited activities. This is observed when the fructose system is induced to high levels and prevented when the fruA/fruB genes are deleted. Overexpression of the fruA and/or fruB genes in the absence of fructose induction during growth also enhances the rates of uptake of other hexoses. The β-galactosidase activities of man, mtl, and gat-lacZ transcriptional fusions and the sugar-specific transphosphorylation activities of these enzyme transporters were not affected either by frustose induction or by fruAB overexpression, showing that the rates of synthesis of the target PTS permeases were not altered. We thus suggest that specific protein-protein interactions within the cytoplasmic membrane regulate transport in vivo (and sometimes the PEP-dependent phosphorylation activities in vitro) of PTS permeases in a physiologically meaningful way that may help to provide a hierarchy of preferred PTS sugars. These observations appear to be applicable in principle to other types of transport systems as well.
Conflict of interest statement
The authors have declared that no competing interests exist.
Figures
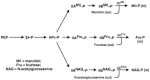
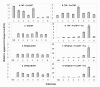
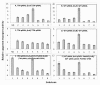
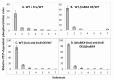
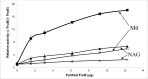
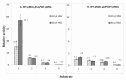
Similar articles
-
Protein-Protein Interactions in the Cytoplasmic Membrane of Escherichia coli: Influence of the Overexpression of Diverse Transporter-Encoding Genes on the Activities of PTS Sugar Uptake Systems.Microb Physiol. 2020;30(1-6):36-49. doi: 10.1159/000510257. Epub 2020 Sep 30. Microb Physiol. 2020. PMID: 32998150 Free PMC article.
-
Dependency of sugar transport and phosphorylation by the phosphoenolpyruvate-dependent phosphotransferase system on membranous phosphatidylethanolamine in Escherichia coli: studies with a pssA mutant lacking phosphatidylserine synthase.Arch Microbiol. 2004 Jan;181(1):26-34. doi: 10.1007/s00203-003-0623-7. Epub 2003 Nov 21. Arch Microbiol. 2004. PMID: 14634719
-
Physiological consequences of the complete loss of phosphoryl-transfer proteins HPr and FPr of the phosphoenolpyruvate:sugar phosphotransferase system and analysis of fructose (fru) operon expression in Salmonella typhimurium.J Bacteriol. 1990 Sep;172(9):5459-69. doi: 10.1128/jb.172.9.5459-5469.1990. J Bacteriol. 1990. PMID: 2203752 Free PMC article.
-
Carbohydrate Transport by Group Translocation: The Bacterial Phosphoenolpyruvate: Sugar Phosphotransferase System.Subcell Biochem. 2019;92:223-274. doi: 10.1007/978-3-030-18768-2_8. Subcell Biochem. 2019. PMID: 31214989 Review.
-
The Escherichia coli mannitol permease as a model for transport via the bacterial phosphotransferase system.J Bioenerg Biomembr. 1993 Dec;25(6):621-6. doi: 10.1007/BF00770249. J Bioenerg Biomembr. 1993. PMID: 8144490 Review.
Cited by
-
Omics analysis coupled with gene editing revealed potential transporters and regulators related to levoglucosan metabolism efficiency of the engineered Escherichia coli.Biotechnol Biofuels Bioprod. 2022 Jan 11;15(1):2. doi: 10.1186/s13068-022-02102-4. Biotechnol Biofuels Bioprod. 2022. PMID: 35418138 Free PMC article.
-
Two Permeases Associated with the Multifunctional CtaP Cysteine Transport System in Listeria monocytogenes Play Distinct Roles in Pathogenesis.Microbiol Spectr. 2023 Jun 15;11(3):e0331722. doi: 10.1128/spectrum.03317-22. Epub 2023 May 18. Microbiol Spectr. 2023. PMID: 37199604 Free PMC article.
-
Protein-Protein Interactions in the Cytoplasmic Membrane of Escherichia coli: Influence of the Overexpression of Diverse Transporter-Encoding Genes on the Activities of PTS Sugar Uptake Systems.Microb Physiol. 2020;30(1-6):36-49. doi: 10.1159/000510257. Epub 2020 Sep 30. Microb Physiol. 2020. PMID: 32998150 Free PMC article.
-
Phosphotransferase System Uptake and Metabolism of the β-Glucoside Salicin Impact Group A Streptococcal Bloodstream Survival and Soft Tissue Infection.Infect Immun. 2020 Sep 18;88(10):e00346-20. doi: 10.1128/IAI.00346-20. Print 2020 Sep 18. Infect Immun. 2020. PMID: 32719156 Free PMC article.
References
Publication types
MeSH terms
Substances
Grants and funding
LinkOut - more resources
Full Text Sources
Molecular Biology Databases
Miscellaneous