Single-Cell RNA-seq Reveals Profound Alterations in Mechanosensitive Dorsal Root Ganglion Neurons with Vitamin E Deficiency
- PMID: 31733517
- PMCID: PMC6864320
- DOI: 10.1016/j.isci.2019.10.064
Single-Cell RNA-seq Reveals Profound Alterations in Mechanosensitive Dorsal Root Ganglion Neurons with Vitamin E Deficiency
Abstract
Ninety percent of Americans consume less than the estimated average requirements of dietary vitamin E (vitE). Severe vitE deficiency due to genetic mutations in the tocopherol transfer protein (TTPA) in humans results in ataxia with vitE deficiency (AVED), with proprioceptive deficits and somatosensory degeneration arising from dorsal root ganglia neurons (DRGNs). Single-cell RNA-sequencing of DRGNs was performed in Ttpa-/- mice, an established model of AVED. In stark contrast to expected changes in proprioceptive neurons, Ttpa-/- DRGNs showed marked upregulation of voltage-gated Ca2+ and K+ channels in mechanosensitive, tyrosine-hydroxylase positive (TH+) DRGNs. The ensuing significant conductance changes resulted in reduced excitability in mechanosensitive Ttpa-/- DRGNs. A highly supplemented vitE diet (600 mg dl-α-tocopheryl acetate/kg diet) prevented the cellular and molecular alterations and improved mechanosensation. VitE deficiency profoundly alters the molecular signature and functional properties of mechanosensitive TH+ DRGN, representing an intriguing shift of the prevailing paradigm from proprioception to mechanical sensation.
Keywords: Molecular Neuroscience; Neuroscience; Transcriptomics.
Copyright © 2019 The Author(s). Published by Elsevier Inc. All rights reserved.
Conflict of interest statement
The authors declare no competing financial interests.
Figures
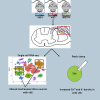
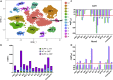
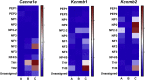
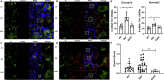
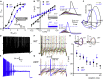
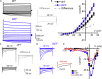
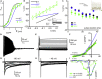
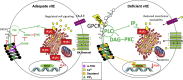
Similar articles
-
Cisplatin Neurotoxicity Targets Specific Subpopulations and K+ Channels in Tyrosine-Hydroxylase Positive Dorsal Root Ganglia Neurons.Front Cell Neurosci. 2022 May 2;16:853035. doi: 10.3389/fncel.2022.853035. eCollection 2022. Front Cell Neurosci. 2022. PMID: 35586548 Free PMC article.
-
Expanding role of vitamin E in protection against metabolic dysregulation: Insights gained from model systems, especially the developing nervous system of zebrafish embryos.Free Radic Biol Med. 2021 Nov 20;176:80-91. doi: 10.1016/j.freeradbiomed.2021.09.016. Epub 2021 Sep 20. Free Radic Biol Med. 2021. PMID: 34555455 Review.
-
MicroRNA-137 and its downstream target LSD1 inversely regulate anesthetics-induced neurotoxicity in dorsal root ganglion neurons.Brain Res Bull. 2017 Oct;135:1-7. doi: 10.1016/j.brainresbull.2017.09.004. Epub 2017 Sep 9. Brain Res Bull. 2017. PMID: 28899795
-
Vitamin E is necessary for zebrafish nervous system development.Sci Rep. 2020 Sep 21;10(1):15028. doi: 10.1038/s41598-020-71760-x. Sci Rep. 2020. PMID: 32958954 Free PMC article.
-
Vitamin E: How much is enough, too much and why!Free Radic Biol Med. 2021 Dec;177:212-225. doi: 10.1016/j.freeradbiomed.2021.10.028. Epub 2021 Oct 23. Free Radic Biol Med. 2021. PMID: 34699937 Review.
Cited by
-
The Impact of LPS on Inflammatory Responses in Alpha-Tocopherol Deficient Mice.Curr Dev Nutr. 2024 Jul 14;8(8):104416. doi: 10.1016/j.cdnut.2024.104416. eCollection 2024 Aug. Curr Dev Nutr. 2024. PMID: 39185446 Free PMC article.
-
Cisplatin Neurotoxicity Targets Specific Subpopulations and K+ Channels in Tyrosine-Hydroxylase Positive Dorsal Root Ganglia Neurons.Front Cell Neurosci. 2022 May 2;16:853035. doi: 10.3389/fncel.2022.853035. eCollection 2022. Front Cell Neurosci. 2022. PMID: 35586548 Free PMC article.
-
Vitamin E Deficiency Disrupts Gene Expression Networks during Zebrafish Development.Nutrients. 2021 Jan 30;13(2):468. doi: 10.3390/nu13020468. Nutrients. 2021. PMID: 33573233 Free PMC article.
-
Intrinsic mechanical sensitivity of mammalian auditory neurons as a contributor to sound-driven neural activity.Elife. 2022 Mar 10;11:e74948. doi: 10.7554/eLife.74948. Elife. 2022. PMID: 35266451 Free PMC article.
-
Physical contact in parent-infant relationship and its effect on fostering a feeling of safety.iScience. 2021 Jun 12;24(7):102721. doi: 10.1016/j.isci.2021.102721. eCollection 2021 Jul 23. iScience. 2021. PMID: 34235413 Free PMC article. Review.
References
-
- Aparicio J.M., Belanger-Quintana A., Suarez L., Mayo D., Benitez J., Diaz M., Escobar H. Ataxia with isolated vitamin E deficiency: case report and review of the literature. J. Pediatr. Gastroenterol. Nutr. 2001;33:206–210. - PubMed
-
- Azzi A. Molecular mechanism of alpha-tocopherol action. Free Radic. Biol. Med. 2007;43:16–21. - PubMed
-
- Azzi A. Many tocopherols, one vitamin E. Mol. Aspects Med. 2018;61:92–103. - PubMed
-
- Bellayou H., Dehbi H., Bourezgui M., Slassi I., Nadifi S. Ataxia with vitamin E deficiency (AVED); an example of the contribution of research in molecular genetic to counselling in Morocco. Pathol. Biol. (Paris) 2009;57:425–426. - PubMed
Grants and funding
LinkOut - more resources
Full Text Sources
Molecular Biology Databases
Miscellaneous