Independent tubulin binding and polymerization by the proline-rich region of Tau is regulated by Tau's N-terminal domain
- PMID: 31699899
- PMCID: PMC6916478
- DOI: 10.1074/jbc.RA119.010172
Independent tubulin binding and polymerization by the proline-rich region of Tau is regulated by Tau's N-terminal domain
Abstract
Tau is an intrinsically disordered, microtubule-associated protein that has a role in regulating microtubule dynamics. Despite intensive research, the molecular mechanisms of Tau-mediated microtubule polymerization are poorly understood. Here we used single-molecule fluorescence to investigate the role of Tau's N-terminal domain (NTD) and proline-rich region (PRR) in regulating interactions of Tau with soluble tubulin. We assayed both full-length Tau isoforms and truncated variants for their ability to bind soluble tubulin and stimulate microtubule polymerization. We found that Tau's PRR is an independent tubulin-binding domain that has tubulin polymerization capacity. In contrast to the relatively weak interactions with tubulin mediated by sites distributed throughout Tau's microtubule-binding region (MTBR), resulting in heterogeneous Tau: tubulin complexes, the PRR bound tubulin tightly and stoichiometrically. Moreover, we demonstrate that interactions between the PRR and MTBR are reduced by the NTD through a conserved conformational ensemble. On the basis of these results, we propose that Tau's PRR can serve as a core tubulin-binding domain, whereas the MTBR enhances polymerization capacity by increasing the local tubulin concentration. Moreover, the NTD appears to negatively regulate tubulin-binding interactions of both of these domains. The findings of our study draw attention to a central role of the PRR in Tau function and provide mechanistic insight into Tau-mediated polymerization of tubulin.
Keywords: Alzheimer's disease; cytoskeleton; fluorescence correlation spectroscopy (FCS); intrinsically disordered protein; microtubule-associated protein (MAP); single-molecule FRET; single-molecule biophysics; tau protein (tau); tauopathy; tubulin polymerization.
© 2019 McKibben and Rhoades.
Conflict of interest statement
The authors declare that they have no conflicts of interest with the contents of this article. The content is solely the responsibility of the authors and does not necessarily represent the official views of the National Institutes of Health
Figures
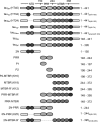
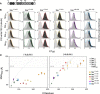
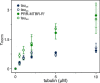
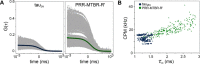
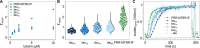
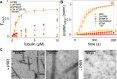
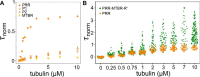
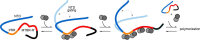
Similar articles
-
Structural insights into the role of the proline rich region in tau function.Structure. 2025 Jan 10:S0969-2126(24)00552-5. doi: 10.1016/j.str.2024.12.017. Online ahead of print. Structure. 2025. PMID: 39826549
-
A functional role for intrinsic disorder in the tau-tubulin complex.Proc Natl Acad Sci U S A. 2016 Dec 13;113(50):14336-14341. doi: 10.1073/pnas.1610137113. Epub 2016 Nov 23. Proc Natl Acad Sci U S A. 2016. PMID: 27911791 Free PMC article.
-
Structural Characterization of Tau in Fuzzy Tau:Tubulin Complexes.Structure. 2020 Mar 3;28(3):378-384.e4. doi: 10.1016/j.str.2020.01.004. Epub 2020 Jan 28. Structure. 2020. PMID: 31995742 Free PMC article.
-
Much More Than a Cytoskeletal Protein: Physiological and Pathological Functions of the Non-microtubule Binding Region of Tau.Front Neurol. 2020 Oct 19;11:590059. doi: 10.3389/fneur.2020.590059. eCollection 2020. Front Neurol. 2020. PMID: 33193056 Free PMC article. Review.
-
Mechanism of tau-induced neurodegeneration in Alzheimer disease and related tauopathies.Curr Alzheimer Res. 2008 Aug;5(4):375-84. doi: 10.2174/156720508785132307. Curr Alzheimer Res. 2008. PMID: 18690834 Review.
Cited by
-
Proteomic Analysis Unveils Expressional Changes in Cytoskeleton- and Synaptic Plasticity-Associated Proteins in Rat Brain Six Months after Withdrawal from Morphine.Life (Basel). 2021 Jul 13;11(7):683. doi: 10.3390/life11070683. Life (Basel). 2021. PMID: 34357055 Free PMC article.
-
The Role of Post-Translational Modifications on the Structure and Function of Tau Protein.J Mol Neurosci. 2022 Aug;72(8):1557-1571. doi: 10.1007/s12031-022-02002-0. Epub 2022 Mar 24. J Mol Neurosci. 2022. PMID: 35325356 Review.
-
The pathogenic R5L mutation disrupts formation of Tau complexes on the microtubule by altering local N-terminal structure.Proc Natl Acad Sci U S A. 2022 Feb 15;119(7):e2114215119. doi: 10.1073/pnas.2114215119. Proc Natl Acad Sci U S A. 2022. PMID: 35135879 Free PMC article.
-
Microtubule-binding core of the tau protein.Sci Adv. 2022 Jul 22;8(29):eabo4459. doi: 10.1126/sciadv.abo4459. Epub 2022 Jul 20. Sci Adv. 2022. PMID: 35857846 Free PMC article.
-
What's in a Gene? The Outstanding Diversity of MAPT.Cells. 2022 Mar 1;11(5):840. doi: 10.3390/cells11050840. Cells. 2022. PMID: 35269461 Free PMC article. Review.
References
Publication types
MeSH terms
Substances
Grants and funding
LinkOut - more resources
Full Text Sources