Metabolic functions of the tumor suppressor p53: Implications in normal physiology, metabolic disorders, and cancer
- PMID: 31685430
- PMCID: PMC7056927
- DOI: 10.1016/j.molmet.2019.10.002
Metabolic functions of the tumor suppressor p53: Implications in normal physiology, metabolic disorders, and cancer
Abstract
Background: The TP53 gene is one of the most commonly inactivated tumor suppressors in human cancers. p53 functions during cancer progression have been linked to a variety of transcriptional and non-transcriptional activities that lead to the tight control of cell proliferation, senescence, DNA repair, and cell death. However, converging evidence indicates that p53 also plays a major role in metabolism in both normal and cancer cells.
Scope of review: We provide an overview of the current knowledge on the metabolic activities of wild type (WT) p53 and highlight some of the mechanisms by which p53 contributes to whole body energy homeostasis. We will also pinpoint some evidences suggesting that deregulation of p53-associated metabolic activities leads to human pathologies beyond cancer, including obesity, diabetes, liver, and cardiovascular diseases.
Major conclusions: p53 is activated when cells are metabolically challenged but the origin, duration, and intensity of these stresses will dictate the outcome of the p53 response. p53 plays pivotal roles both upstream and downstream of several key metabolic regulators and is involved in multiple feedback-loops that ensure proper cellular homeostasis. The physiological roles of p53 in metabolism involve complex mechanisms of regulation implicating both cell autonomous effects as well as autocrine loops. However, the mechanisms by which p53 coordinates metabolism at the organismal level remain poorly understood. Perturbations of p53-regulated metabolic activities contribute to various metabolic disorders and are pivotal during cancer progression.
Keywords: Cancer; Metabolism; Normal tissue homeostasis; p53.
Copyright © 2019 The Authors. Published by Elsevier GmbH.. All rights reserved.
Figures
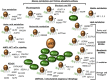
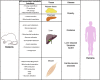
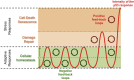
Similar articles
-
The crisscross between p53 and metabolism in cancer.Acta Biochim Biophys Sin (Shanghai). 2023 Jun 19;55(6):914-922. doi: 10.3724/abbs.2023109. Acta Biochim Biophys Sin (Shanghai). 2023. PMID: 37337630 Free PMC article. Review.
-
Tumor suppressor p53 and its mutants in cancer metabolism.Cancer Lett. 2015 Jan 28;356(2 Pt A):197-203. doi: 10.1016/j.canlet.2013.12.025. Epub 2013 Dec 24. Cancer Lett. 2015. PMID: 24374014 Free PMC article. Review.
-
Homeostatic functions of the p53 tumor suppressor: regulation of energy metabolism and antioxidant defense.Semin Cancer Biol. 2009 Feb;19(1):32-41. doi: 10.1016/j.semcancer.2008.11.005. Epub 2008 Dec 3. Semin Cancer Biol. 2009. PMID: 19101635 Free PMC article. Review.
-
The p53 Pathway and Metabolism: The Tree That Hides the Forest.Cancers (Basel). 2021 Jan 4;13(1):133. doi: 10.3390/cancers13010133. Cancers (Basel). 2021. PMID: 33406607 Free PMC article. Review.
-
Regulation and function of p53: A perspective from Drosophila studies.Mech Dev. 2018 Dec;154:82-90. doi: 10.1016/j.mod.2018.05.007. Epub 2018 May 23. Mech Dev. 2018. PMID: 29800619 Review.
Cited by
-
P53 Alleviates the Progression of Periodontitis by Reducing M1-type Macrophage Differentiation.Inflammation. 2024 Aug;47(4):1170-1184. doi: 10.1007/s10753-024-01968-w. Epub 2024 Feb 6. Inflammation. 2024. PMID: 38319542 Free PMC article.
-
Cisatracurium inhibits the growth and induces apoptosis of ovarian cancer cells by promoting lincRNA-p21.Bioengineered. 2021 Dec;12(1):1505-1516. doi: 10.1080/21655979.2021.1916271. Bioengineered. 2021. PMID: 33944652 Free PMC article.
-
Editorial: Genomic alteration landscapes of aging, metabolic disorders, and cancer: Emerging overlaps and clinical importance.Front Genet. 2023 Jan 6;13:1102953. doi: 10.3389/fgene.2022.1102953. eCollection 2022. Front Genet. 2023. PMID: 36685844 Free PMC article. No abstract available.
-
Targeted Protein Degradation: An Important Tool for Drug Discovery for "Undruggable" Tumor Transcription Factors.Technol Cancer Res Treat. 2022 Jan-Dec;21:15330338221095950. doi: 10.1177/15330338221095950. Technol Cancer Res Treat. 2022. PMID: 35466792 Free PMC article.
-
UBE2M Drives Hepatocellular Cancer Progression as a p53 Negative Regulator by Binding to MDM2 and Ribosomal Protein L11.Cancers (Basel). 2021 Sep 29;13(19):4901. doi: 10.3390/cancers13194901. Cancers (Basel). 2021. PMID: 34638383 Free PMC article.
References
-
- Kruiswijk F., Labuschagne C.F., Vousden K.H. p53 in survival, death and metabolichealth: a lifeguard with a licence to kill. Nature Reviews Molecular Cell Biology. 2015;16(7):393–405. - PubMed
Publication types
MeSH terms
Substances
LinkOut - more resources
Full Text Sources
Medical
Research Materials
Miscellaneous